Page snapshot: An overview of the fossil record of grasses and the development of grasslands from the Cretaceous to the recent.
Topics covered on this page: Introduction; Cretaceous (145 to 66 million years ago); Paleogene (66 to 23 million years ago); Neogene (23 to 2.6 million years ago); Pleistocene to recent (2.6 million years ago to present); Resources.
Credits: Funded by the National Science Foundation. Any opinions, findings, and conclusions or recommendations expressed in this material are those of the author(s) and do not necessarily reflect the views of the National Science Foundation. This page incorporates content prepared for a revised manuscript of the Teacher-Friendly Guide to the Evolution Maize (Carlyn Buckler, Dhyan Palanichamy, and Andrielle Swaby, 2019). Additional content and revisions by Elizabeth J. Hermsen and Naomi Schulberg (2022-2023).
Updates: Page last updated August 28, 2023.
Image above: The Ukok Plateau in Siberia, Russia. The Altai Mountains region, where the Ukok Plateau is located, is similar to (and may be a remnant of) the once widespread Pleistocene mammoth-steppe (steppe-tundra) grassland biome. Photo by Kobsev (Wikimedia Commons, Creative Commons Attribution 2.5 Generic license).
Introduction
The fossil record of grasses begins in the late Early Cretaceous (about 145 to 100.5 million years ago) and extends to the recent. It includes macrofossils (fossils that can be seen without a microscope), although most of the record consists of microfossils, such as pollen, phytoliths, and epidermal fragments. In the Cretaceous (about 145 to 66 million years ago) and Paleogene (about 66 to 23 million years ago), the record is sparse, although it does tell us two things: 1) grasses were present, even if they were not as dominant in some ecosystems as they are today, and 2) the major groups of grasses were diversifying as early as the Cretaceous. In the Neogene (about 23 to 2.6 million years ago), the fossil record of grasses explodes, reflecting changes in global climate that favored the expansion of open habitats. Data from grass fossils is supplemented by data from animal fossils and isotopes to give a picture of the evolution of grasslands through time.
Today, much of the world is covered by grasslands and/or tropical savannas (a type of habitat with scattered trees among grasses), including large parts of every continent except Antarctica and Europe (which still has grasslands in the southeast).
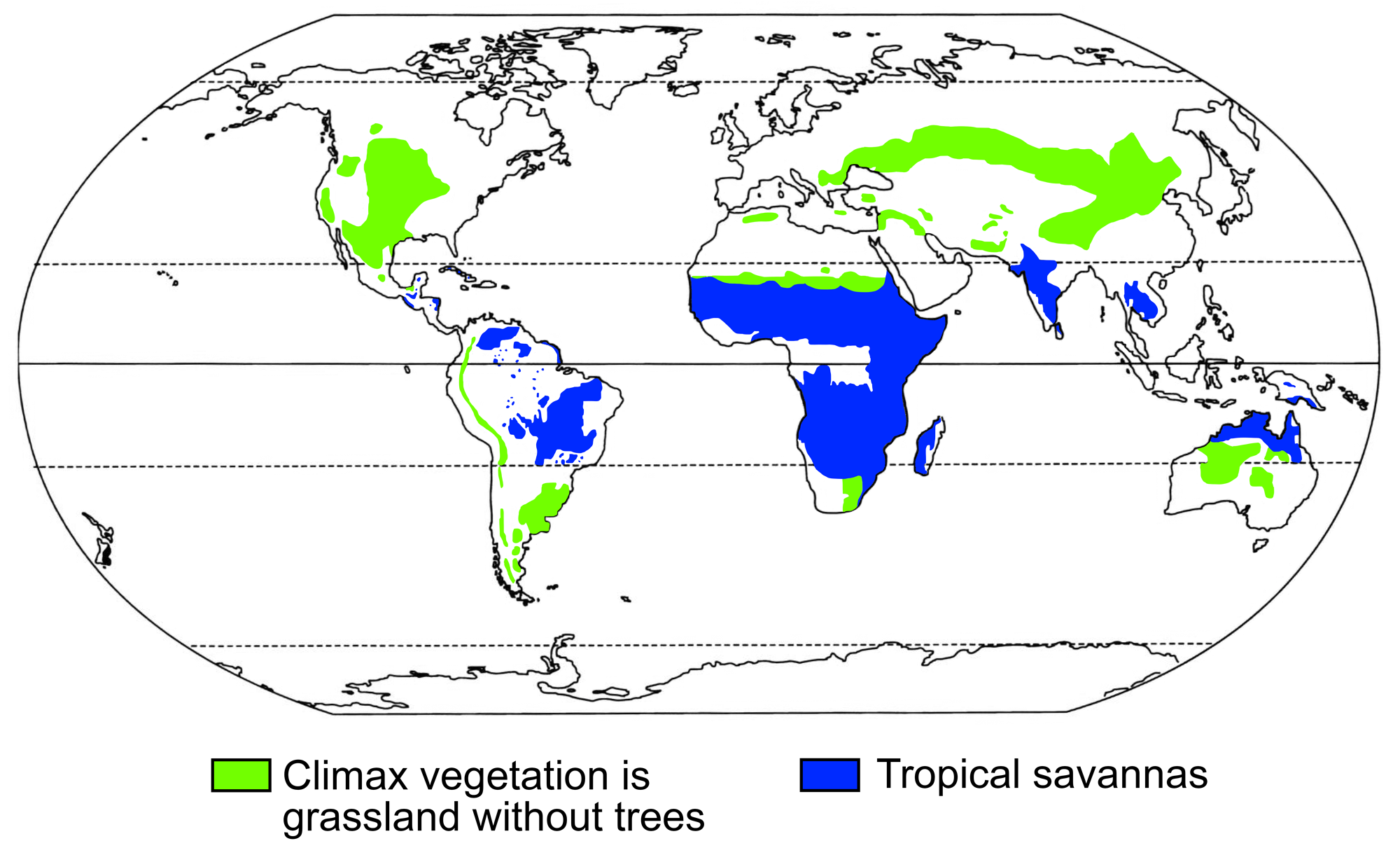
Modern distribution of grasslands (meaning biomes in which the climax vegetation is grass-dominated and lacks tress) and tropical savannas. Modified from figure 1 in Jacobs et al. (1999) Annals of the Missouri Botanical Garden 86: 590-643 (Biodiversity Heritage Library, Creative Commons-NonCommercial-ShareAlike 3.0 Unported license, image cropped, resized, reshaded and relabeled).
Cretaceous (145 to 66 million years ago)
Grasses evolved into a landscape ruled by dinosaurs, conifers, cycads, and flowering plants. Fossilized pollen grains reveal that angiosperms (seed-bearing plants that have flowers) diverged from gymnosperms (seed-bearing plants that do not have flowers) by at least the Early Cretaceous, which began 145 million years ago. The earliest credible angiosperm macrofossils, like Archaefructus liaoningensis and Montsechia vidalii, date to the Early Cretaceous (as old as about 130 to 125 million years ago). Angiosperms continued to diversify throughout the Cretaceous period, co-evolving with the insects that pollinated them. By the end of the Cretaceous, they had begun to dominate the landscape.
Grasses (the Grass Family or Poaceae) descend from a single common ancestor. The first grasses are thought to have evolved in the Early Cretaceous, based on fossil evidence. At that time, the Earth's southern continents—South America, Antarctica, Africa, Australia, and the Indian subcontinent—were joined together in a supercontinent called Gondwana; the northern continents—North America, Europe, and Asia (minus India)—were grouped into a supercontinent called Laurasia.
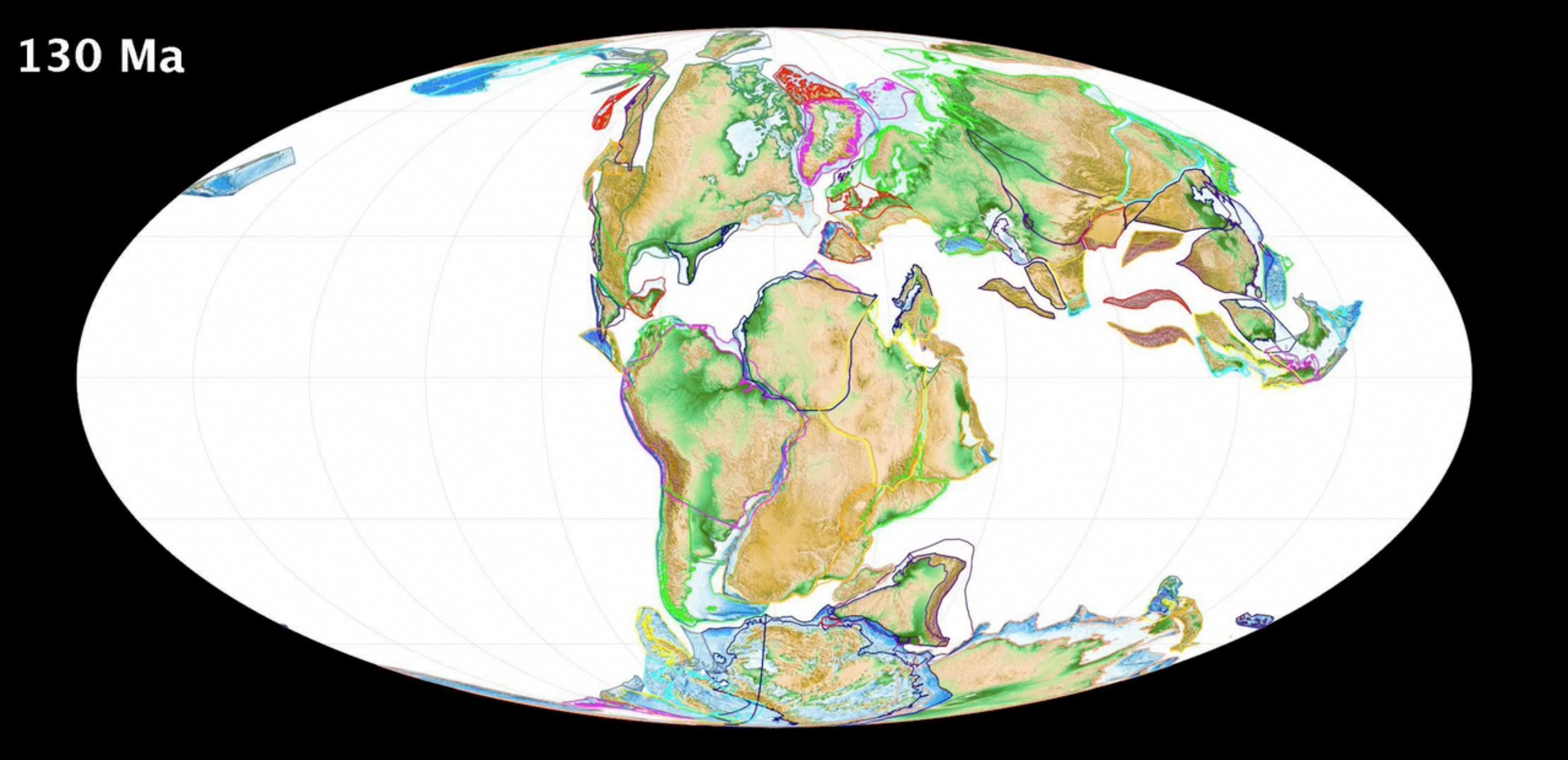
A map of the Earth 130 million years ago, in the Early Cretaceous. Image from Wright, N., Zahirovic, S., Müller, R. D., and Seton, M. Towards community-driven paleogeographic reconstructions: integrating open-access paleogeographic and paleobiology data with plate tectonics, Biogeosciences, 10, 1529-1541, doi:10.5194/bg-10-1529-2013, 2013.
The oldest grass fossils date to the late Early Cretaceous and are about 113 to 101 million years old. These fossils are fragments of grass-like epidermis and phytoliths that were found on the teeth of a hadrosaur (duck-billed dinosaur) from China. These early fragments are thought to represent basally diverging grasses, and could even represent completely extinct groups of early grasses.
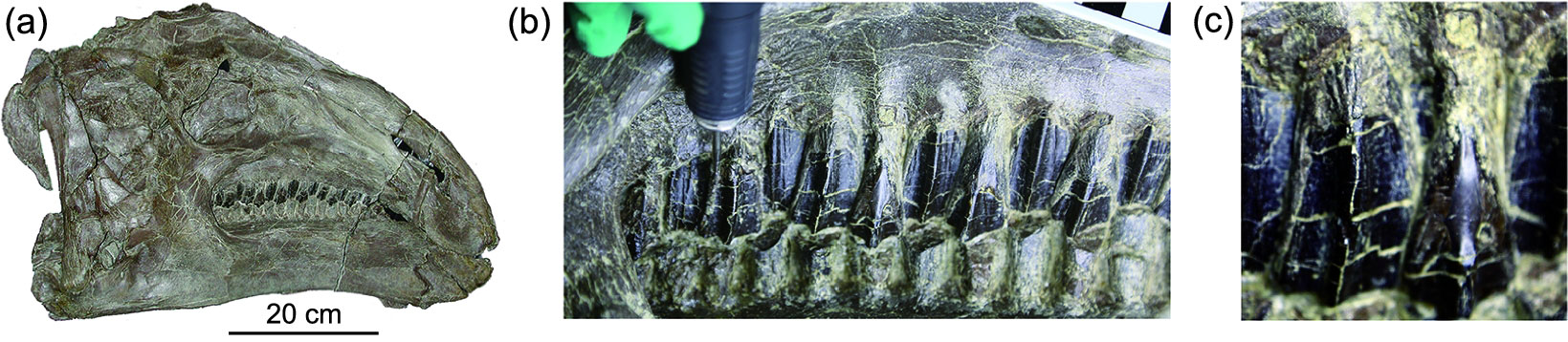
Views of a hadrosaur skull and teeth, Early Creatceous, China. Phytoliths were sampled from this skull in the position shown with the probe in image b. Source: Figure 1 from Wu et al. (2018) National Science Review 5: 721-727 (Creative Commons Attribution 4.0 International license).
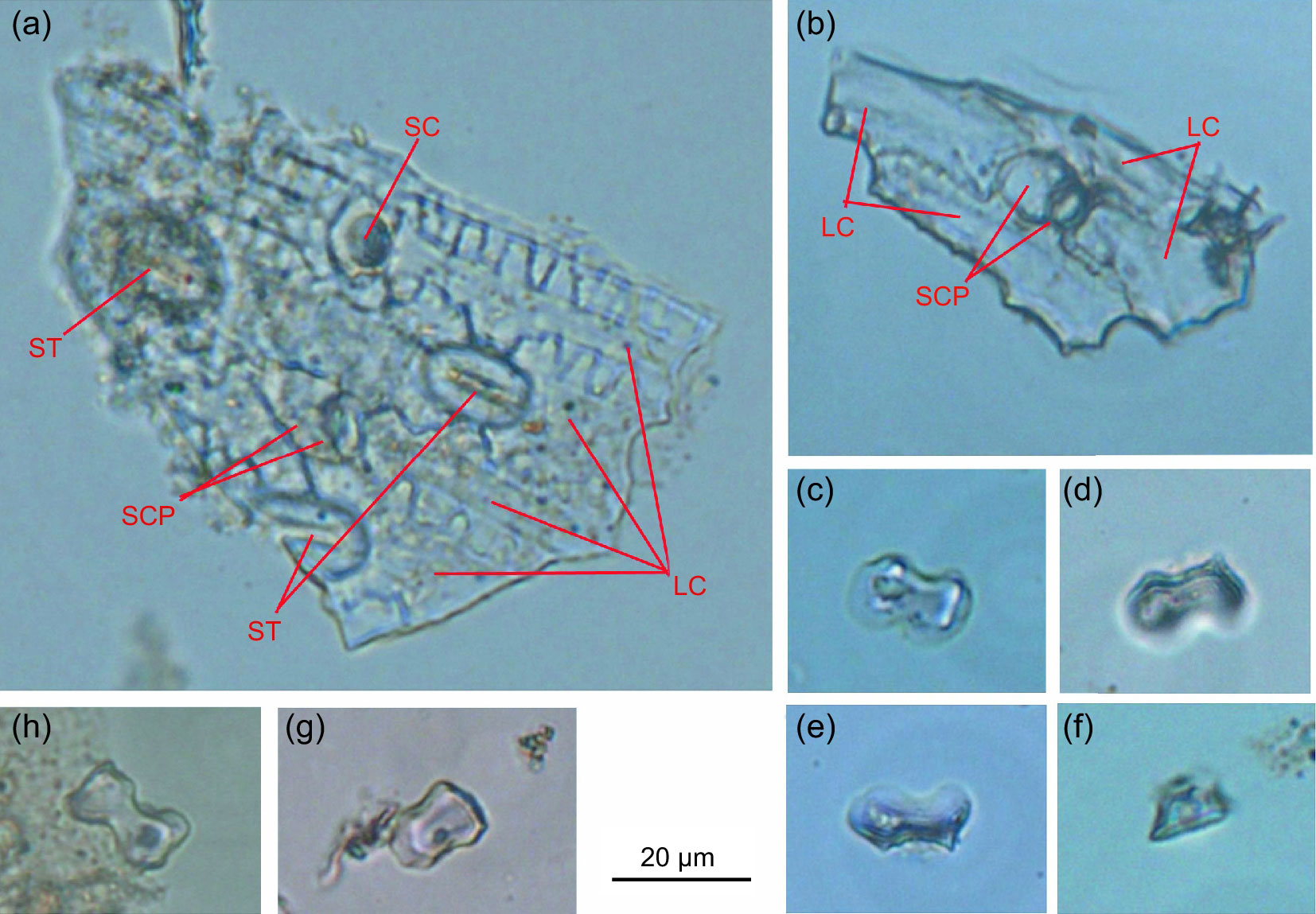
Phytoliths isolated from a hadrosaur skull, Early Creatceous, China. Images a and b show epidermal fragments, images c to e to h show isolated phytoliths. Labels: LC = long cell; SC = short cell; SCP = short cell pair; ST = stoma. Source: Figure 2 from Wu et al. (2018) National Science Review 5: 721-727 (Creative Commons Attribution 4.0 International license).
The oldest known grass macrofossils come from Late Cretaceous Burmese amber and are estimated to be about 110 to 94 million years old. These fossils include an unnamed grass spikelet infected with an ergot-like fungus and fossils assigned to the genus Programinis, including a spikelet (Programinis burmitis) and a leaf fragment (Programinis laminatus). Grass-like silica bodies (phytoliths) have been found in the leaf fragment, cementing its identity as a grass. As with the grass fossils from China, these fossils may represent completely extinct grasses.
The ancestor of all living grasses is thought to have lived on Gondwana, and by the Late Cretaceous (100.5 to 66 million years ago) much of the grass fossil record is from Gondwanan continents. Grass phytoliths found in the coprolites (fossilized dung) of sauropod dinosaurs (long-necked dinosaurs) dating from the very end of the Cretaceous (72 to 66 million years ago) have been found in India. Some of the phytoliths came from grasses in the BOP and PACMAD clades (the two largest groups of grasses), indicating that grasses had already partially diversified by the end of the Cretaceous.
Possible grass pollen (pollen assigned to the genus Monoporites) has been found in Africa, India, and South America near the end of the Cretaceous, indicating that grasses were present on several continents by that time.
Paleogene (66 to 23 million years ago)
During the Paleocene (66 to 55 million years ago) to Eocene (about 55 to 34 million years ago) epochs, Earth's continents were getting closer to their present positions, although land bridges in the North Atlantic and North Pacific connected Eurasia and North America, and land bridges across the Southern Ocean connected Antarctica to both Australia and South America. (Note that these "land bridges" may have been interrupted by water, but the land masses were close enough that plants could disperse among them more easily than they can today.) Thus, plants were still able to expand their ranges across these adjoining continents. The Earth's global temperature was very warm in the late Paleocene to early Eocene, and cold-sensitive plants and animals could be found at high latitudes because the Earth's poles were much warmer than they are today.
Following their peak in the early Eocene, global temperatures cooled into the beginning of the Oligocene epoch (34 to 23 million years ago). Grasslands began to develop as early as the Eocene in North America, with open grasslands starting to replace forests beginning in the late Oligocene (about 26 million years ago). Evidence suggests that this change was driven by drying of the climate in North America, particularly during the winter season. The shift to grasslands took place later in Africa and Australia.
Grass pollen records are sparse during the Paleocene, occurring in South America, Africa, and India. Eocene pollen records indicate that grasses were widely distributed, found in North America, South America, Africa, Australia, Europe, and the eastern part of Asia. Phytolith records come mostly from the Americas and Turkey, and indicate that bamboos, pooids (cool-season grasses), and PACMADs were present.
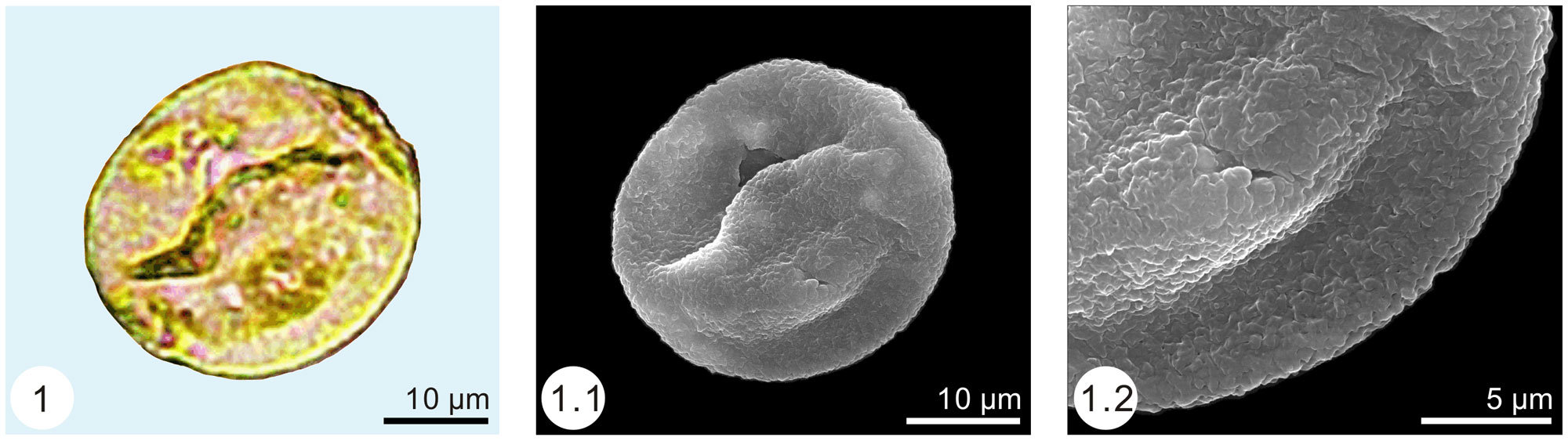
Three views of fossil grass pollen (Graminidites) from the Eocene of China. From left to right: Whole pollen grain under a light microscope, whole pollen grain under a scanning electron microscope, and detail of the pollen wall under a scanning electron microscope. Source: From figure 5 in Zheng et al. (2016) PLoS ONE 11(6): e0157802 (Creative Commons Attribution 4.0 International license, image cropped and resized).
Grass macrofossils from the Eocene to Oligocene are relatively rare. Compression fossils of grass spikelets containing pollen are known from the lower Eocene Wilcox Formation in Tennessee, U.S.A. Another report from North America is from the late Eocene Florissant flora of Colorado, U.S.A.; this report is of fruits assigned to the species Stipa florissantii. Stipa is a modern genus, and it is unclear if the Florissant grass really belongs within it. Additional scattered reports are known from the western U.S. and likely require further investigation.
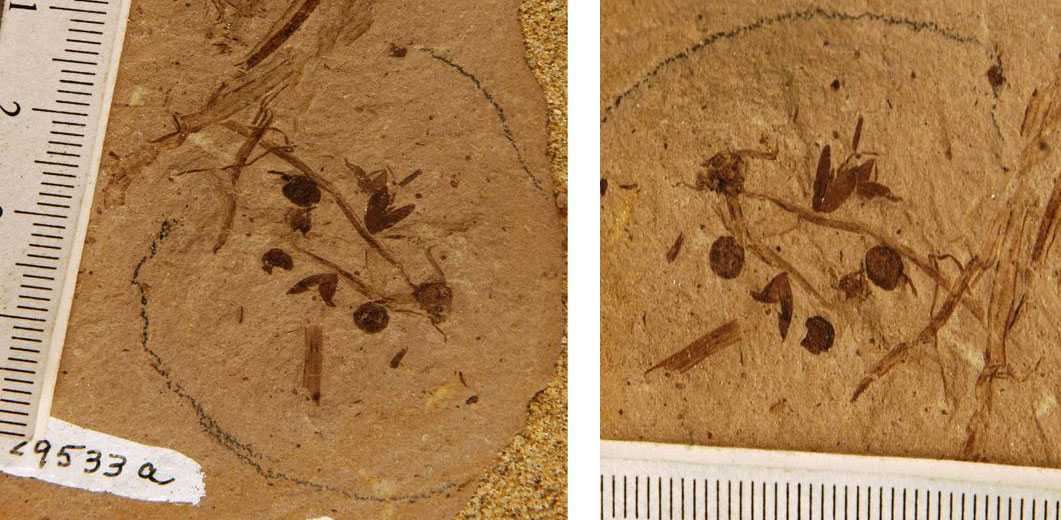
Part and counterpart of a fossil grass (Grass Family/Poaceae) from the Eocene to Oligocene Beaverhead Basins, Montana, U.S.A. Photos of YPM PB 029533 by Division of Paleobotany, Yale Peabody Museum, 2015 (GBIF.org, CC0 1.0 Universal/public domain dedication).
In Europe, the spikelet Eograminis balticus has been found in Baltic amber (a European deposit), which probably dates to the Eocene. Eograminis is similar to moor grass (Molinia) in subfamily Arundinoideae of the PACMAD clade; modern members of this subfamily include the common reed (Phragmites australis) and the giant reed (Arundo donax). Other possible grass fossils found in Baltic amber are now considered doubtful.
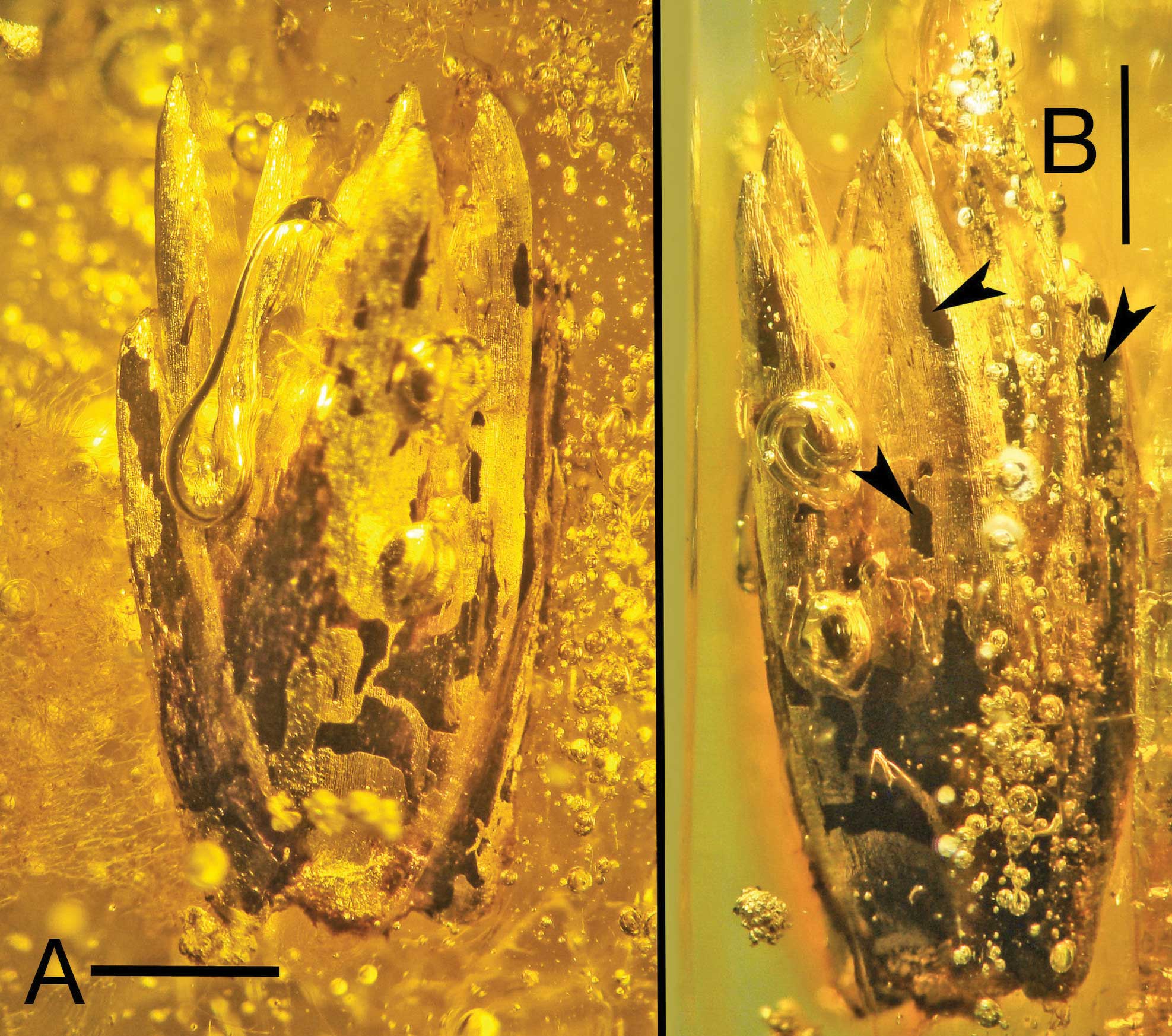
Two views of the spikelet of Eograminis balticus, Eocene Baltic Amber. Left scale bar = 0.4 mm, right scale bar = 1 mm. Source: Figure 2 from Poinar and Soreng (2021) International Journal of Plant Sciences 182: 808-816 (Creative Commons Attribution-NonCommercial 4.0 International license, image resaved with more compression).
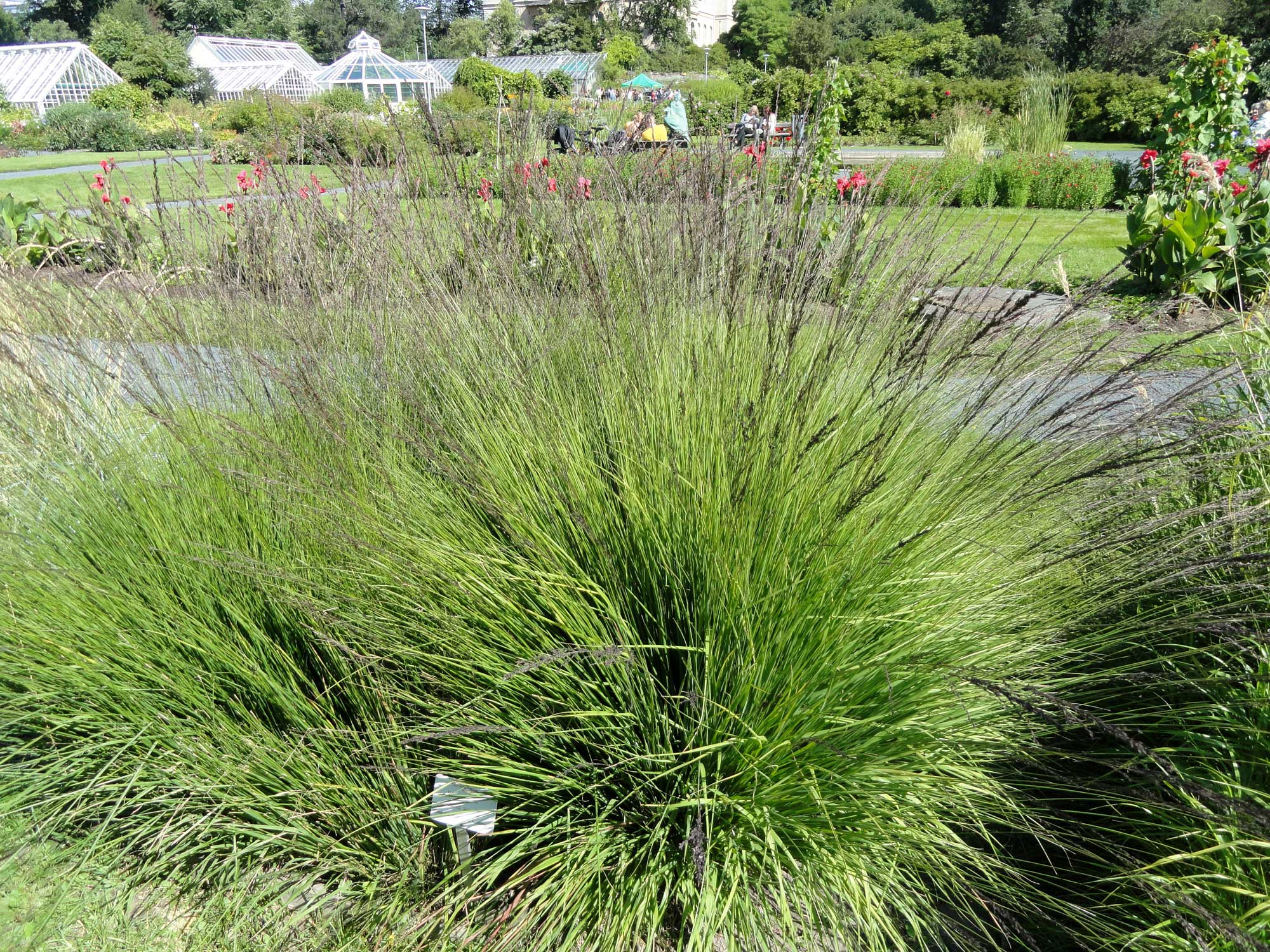
Purple moor grass (Molinia caerulea) cultivated in a botanical garden, Helsinki, Finland. Photo by Daderot (Wikimedia Commons, Creative Commons CC0 1.0 Universal Public Domain Dedication).
In South America, a macrofossil of Chusquea, a type of bamboo that occurs today from Mexico to South America and in the Caribbean, has since been identified as a conifer branch with leaves.
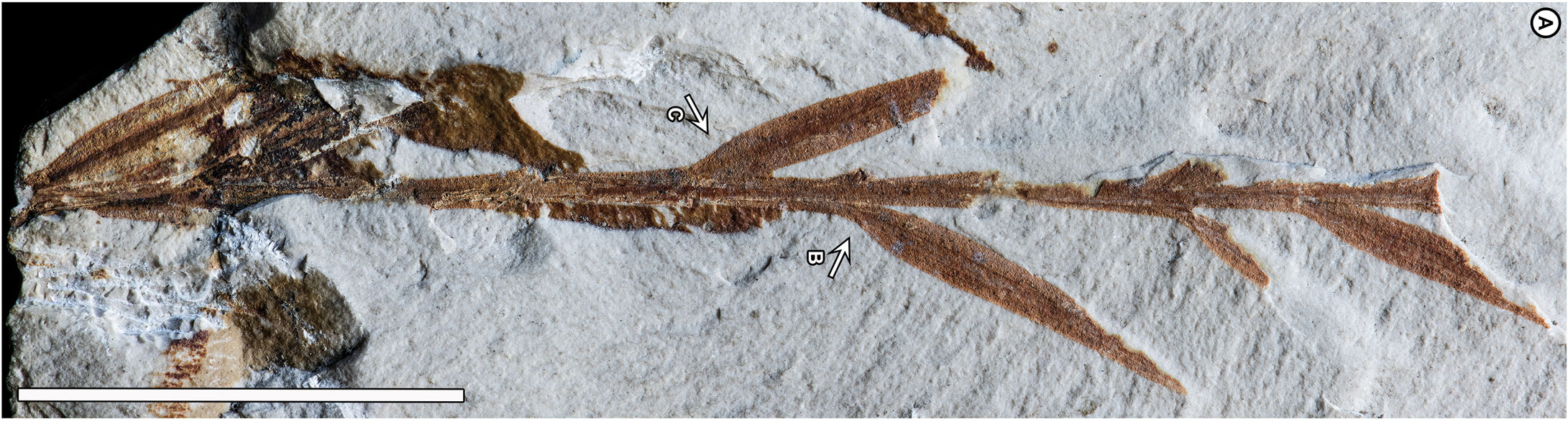
A branch and leaves of the conifer Retrophyllum oxyphyllum from the Eocene of Patagonia, Argentina, formerly thought to have been a fossil bamboo. Scale bar = 2 mm. Source: Figure 1 from Wilf (2020) PhytoKeys 139 (Creative Commons Attribution 4.0 International license, image cropped).
Neogene (23 to 2.6 million years ago)
Spread of grasslands
The fossil record of grasses is much richer in the Neogene (23 million years ago to 2.6 million years ago), reflecting changes in global climate, particularly global cooling and the drying of continental interiors. Events like the initiation of the Antarctic Circumpolar Current near the Eocene-Oligocene boundary, the uplift of the Himalayas in the Neogene, and the closure of the Isthmus of Panama about 3.5 million years ago contributed to global cooling. In South America, the uplift of the Andes mountain range played an important regional role, casting a rain shadow to the east.
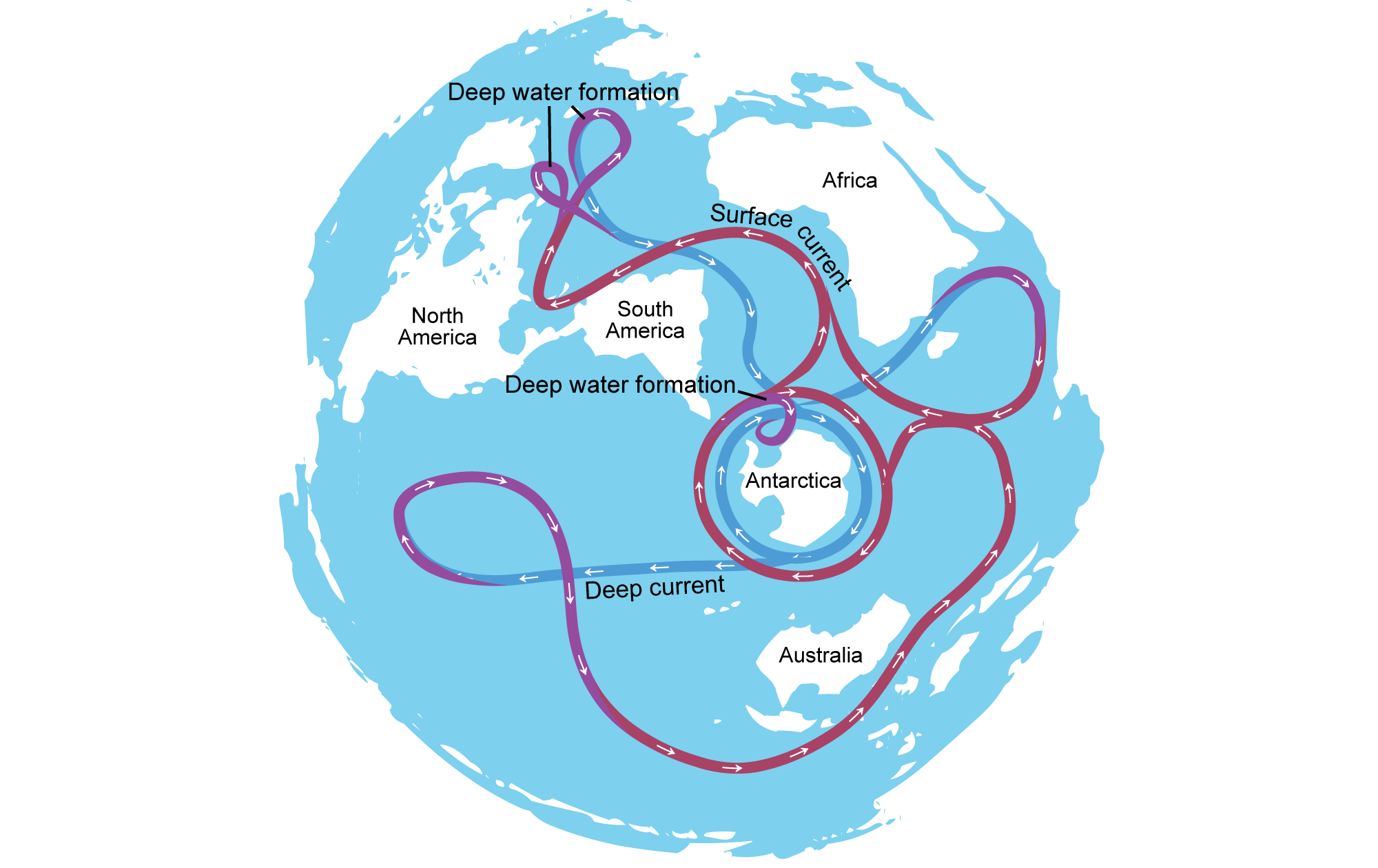
Illustration showing the circulation of water around the world's oceans today. The Antarctic Circumpolar Current is the current that encircles Antarctica. Modified from a diagram by Avsa (Wikimedia Commons, Creative Commons Attribution-ShareAlike 3.0 Unported license, image labels modified and image resized).
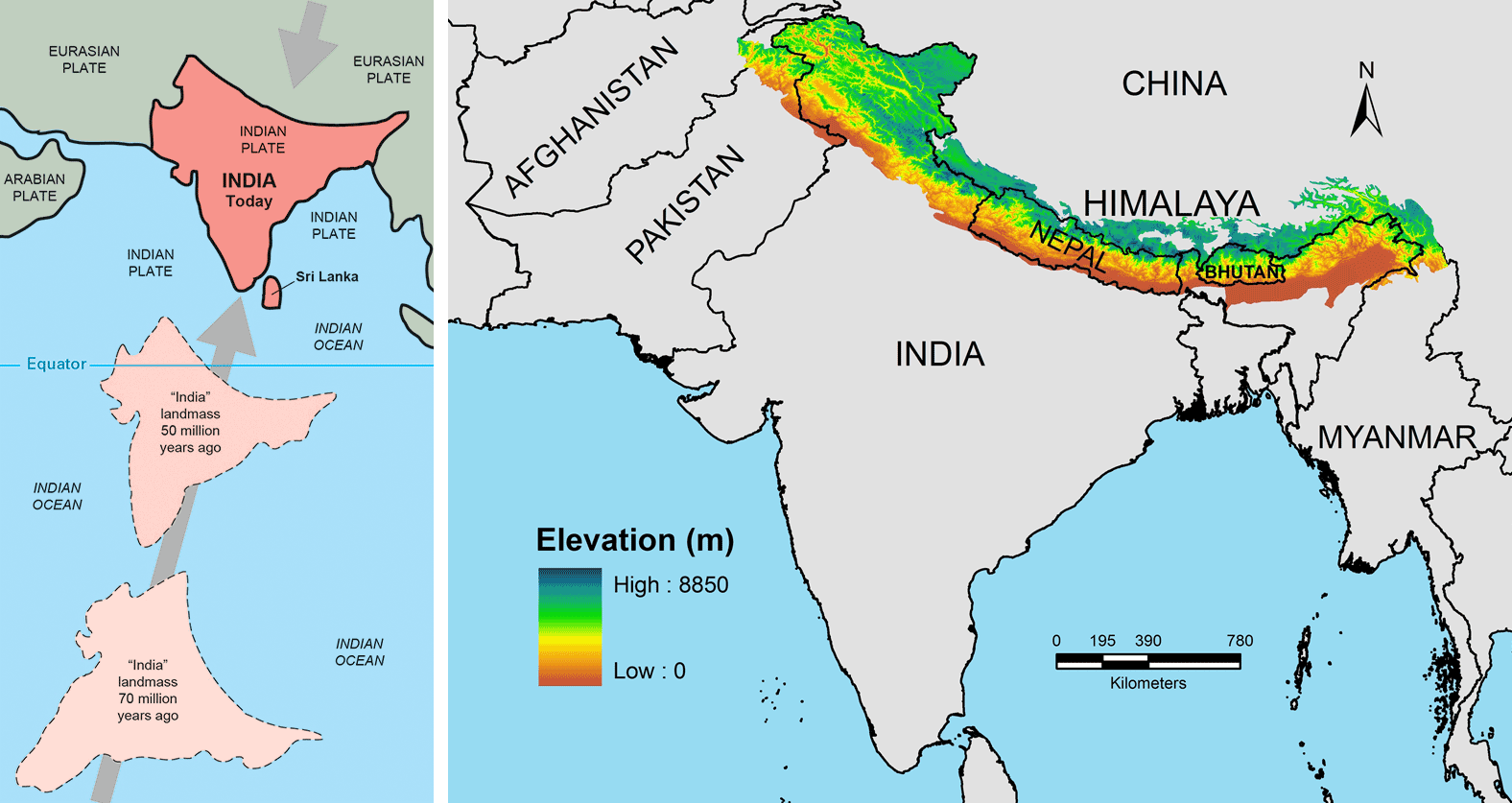
Left: The changing positions of the Indian subcontinent in the Late Cretaceous (70 million years ago), the Paleogene (50 million years ago) and today. Illustration from Kious and Tilling (1996) This Dynamic Earth: the Story of Plate Tectonics (USGS). Right: Map of the modern Himalayan Mountains in Asia. These mountains formed when the Indian subcontinent collided with Asia. Figure 1 from Manish and Pandit (2018) PeerJ 6:e5919 (Creative Commons Attribution 4.0 International license, image resized).
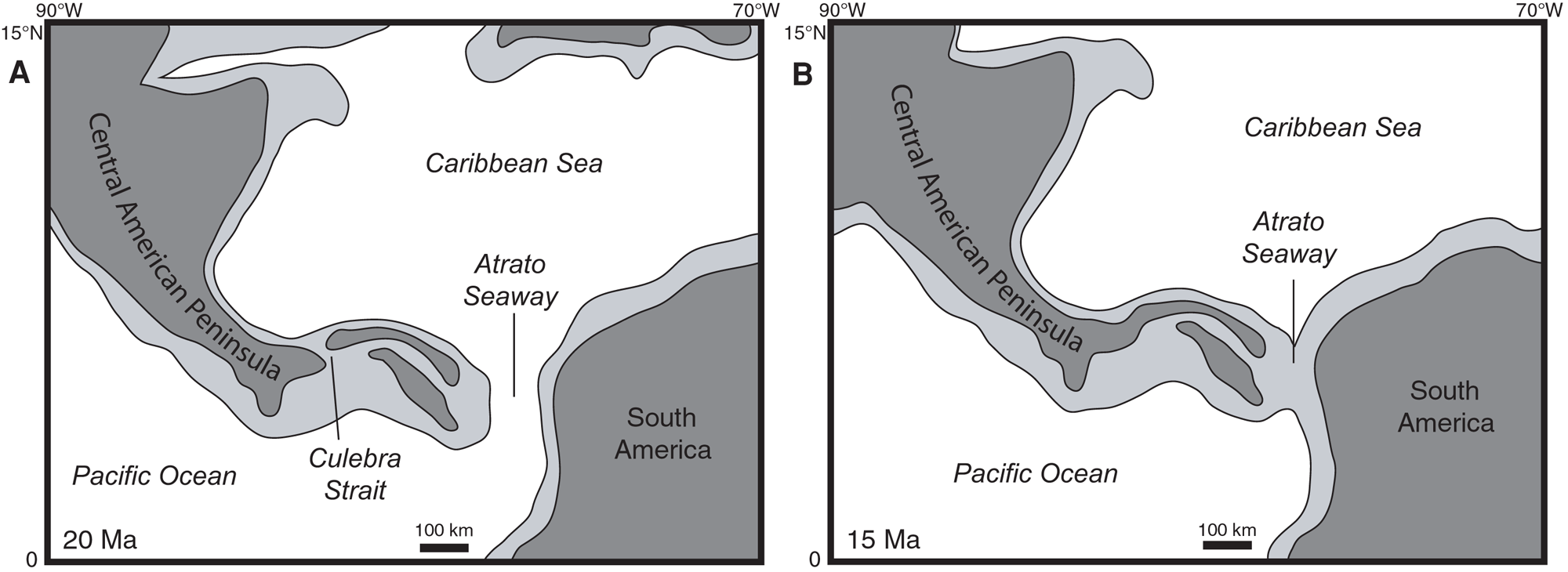
Maps showing the progressive closure of the Isthmus of Panama at 20 million years ago (A) and 15 million years ago (B). Dark gray is land, white and light gray are submerged areas. Modified from Figure 11 in Kirby et al. (2011) PLoS ONE 3(7): e2791 (Creative Commons Attribution license, image reorganized and resized).
Grasses thrived in these new drier and cooler habitats, dominating areas that were now too dry to sustain forests. Open, grass-dominated habitats began to appear, and grassland biomes became widespread in the late Neogene. During the later part of the Neogene, there was also a shift to increasing prevalence of C4 grasses in grasslands, as evidenced by stable carbon isotope data, mostly from paleosols and ungulate tooth enamel. C4 grasses thrive in drier and hotter climates than cool-season (C3) grasses.
The first evidence of C4-dominated grasslands is from Kenya and Uganda in eastern Africa, at about 21 to 16 million years ago. The inference that C4 grasses were present comes from phytoliths, stable carbon isotope analyses, and other types of data. Most global evidence for widespread dominance of C4 grasslands is less than 10 million years old.
There are various hypotheses explaining the shift from the predominance of C3 to C4 grasses in the Neogene. One hypothesis is that the relatively frequent fires associated with grasses (and, specifically, C4 grasses) acted as a positive feedback mechanism, thereby further increasing C4 grass abundance. Grassland-fire feedbacks are generally seen as a major stimulator of C4 grass-dominated ecosystems and function to maintain and even spread grasslands today.
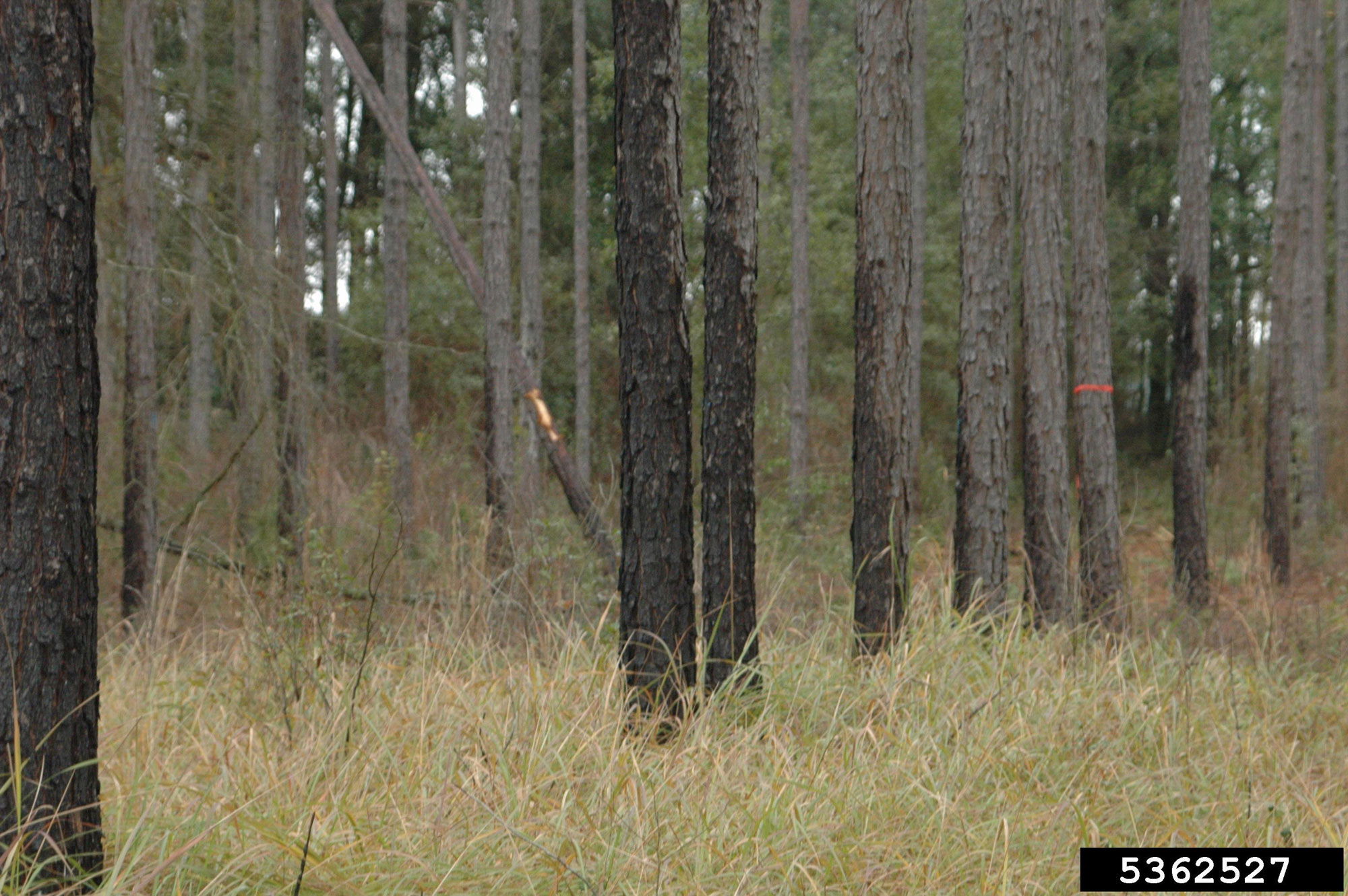
Cogongrass (Imperata cylindrica), a C4 grass that is invasive (weedy) in parts of the world today. This grass is fire-adapted and burns at high temperatures, which helps it to gain and maintain dominance in certain ecosystems. The scorch marks on the trees above were made by cogongrass burning. Photo by Carey Minteer, University of Florida (Bugwood.org photo UGA536527, Creative Commons Attribution 3.0 United States license).
A decline in atmospheric carbon dioxide (CO2) occurred around the same time as this vegetation shift. While scientists previously believed that this decline was caused by an increase in C4 photosynthesis that drew down the amount of carbon dioxide in the Earth's atmosphere, they now more commonly think that the drop in atmospheric carbon dioxide caused a shift to more C4 photosynthesis. C4 photosynthesis shuttles carbon dioxide into specialized bundle sheath cells in the leaf, which reduces photorespiration, the wasteful use of an oxygen molecule rather than a carbon dioxide molecule during the photosynthetic process. C4 plants are therefore more efficient at using of carbon dioxide during photosynthesis than C3 plants, and lower concentrations of atmospheric carbon dioxide may have favored plants with C4 photosynthesis.
During the Pliocene (5.3 to 2.6 million years ago), the climate became increasingly cold and arid, facilitating the expansion of grasslands in low-latitude areas. The Pliocene saw further increases in the expansion of C4-dominated vegetation on almost all continents.
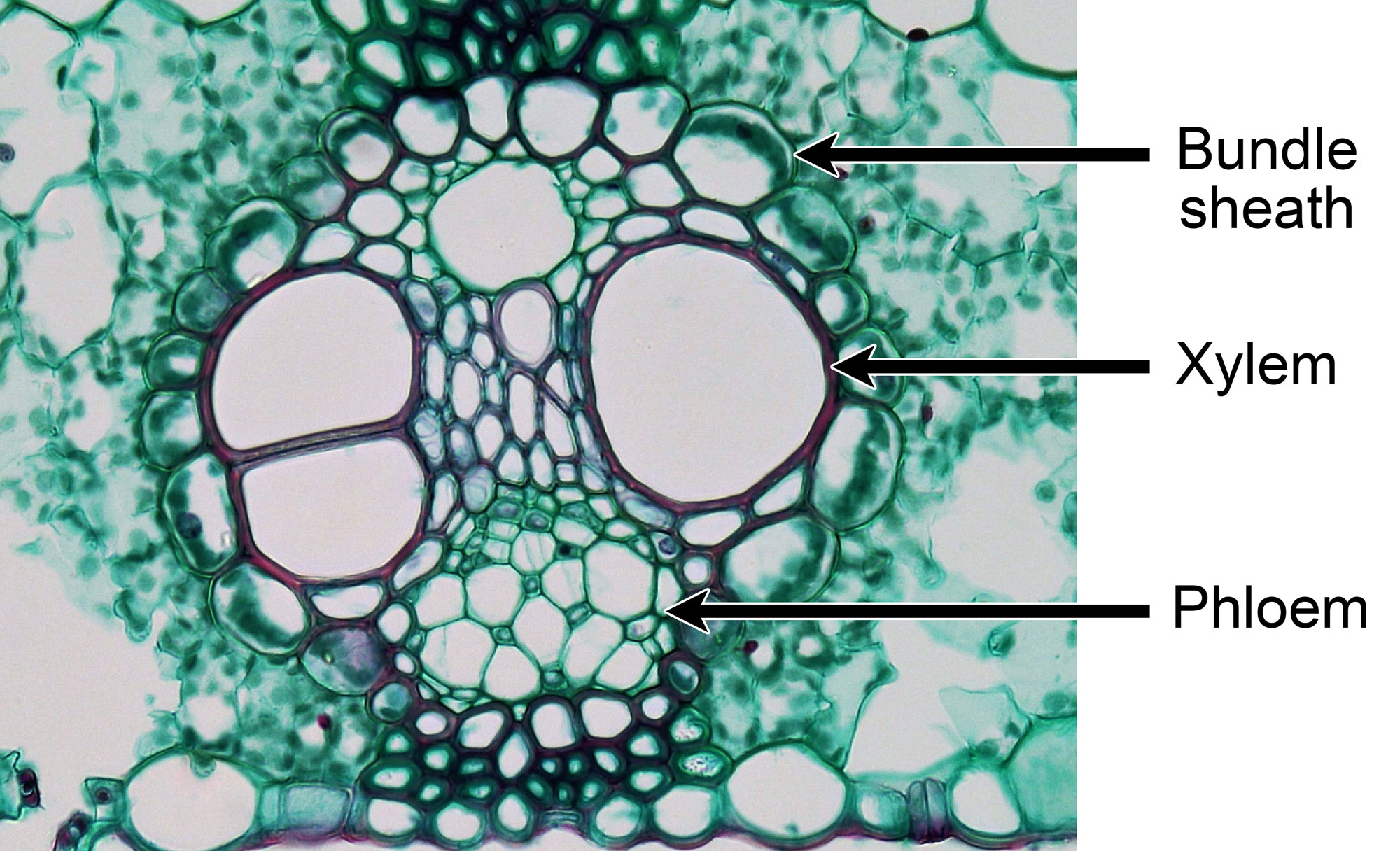
Fixed and stained cross section of a leaf of maize (corn, Zea mays), an extant C4 grass, showing a large vascular bundle encircled by a single-layered bundle sheath that has cells containing chloroplasts. The bundle sheath cells play a key role in the process of photosynthesis in C4 grasses, helping to reduce photorespiration. Photo by Berkshire Community College (flickr, CC0 1.0 Univerisal/public domain dedication).
Neogene grass fossil record
The Neogene fossil record of grasses is significantly richer than the Cretaceous to Paleogene records in the abundance of reports. Fossil evidence of grasses comes from from every continent, including Antarctica. While pollen and phytolith records remain important, multiple macrofossil records are also documented from western North America (more specifically, the United States west of the Mississippi river), Europe, and eastern Africa, with a few additional records from South America, western Africa, eastern North America, and even northern Canada. Many Neogene records are simply assigned to the Grass Family (Poaceae), although some can be assigned to large subgroups (such as PACMADs), subfamilies, or even genera of grasses.
An interesting Miocene (23 to 5.3 million years ago) record comes from the Dominican Republic amber Lagerstattë (fossil deposit with exceptional preservation). It is a grass spikelet (Pharus primuncinatus) assigned to Pharus, a living genus of grasses sometimes called stalkgrasses that are found today in the subtropical to tropical Americas. The fossil spikelet has hook-like hairs and was preserved in the same piece of amber as a few strands of mammal hair, providing plausible evidence of epizoochory (dispersal of a seed or fruit on the outside of an animal's body, for example by gripping a mammal's hair). A spikelet of an extinct bamboo (Alarista succina) comes from the same deposit.
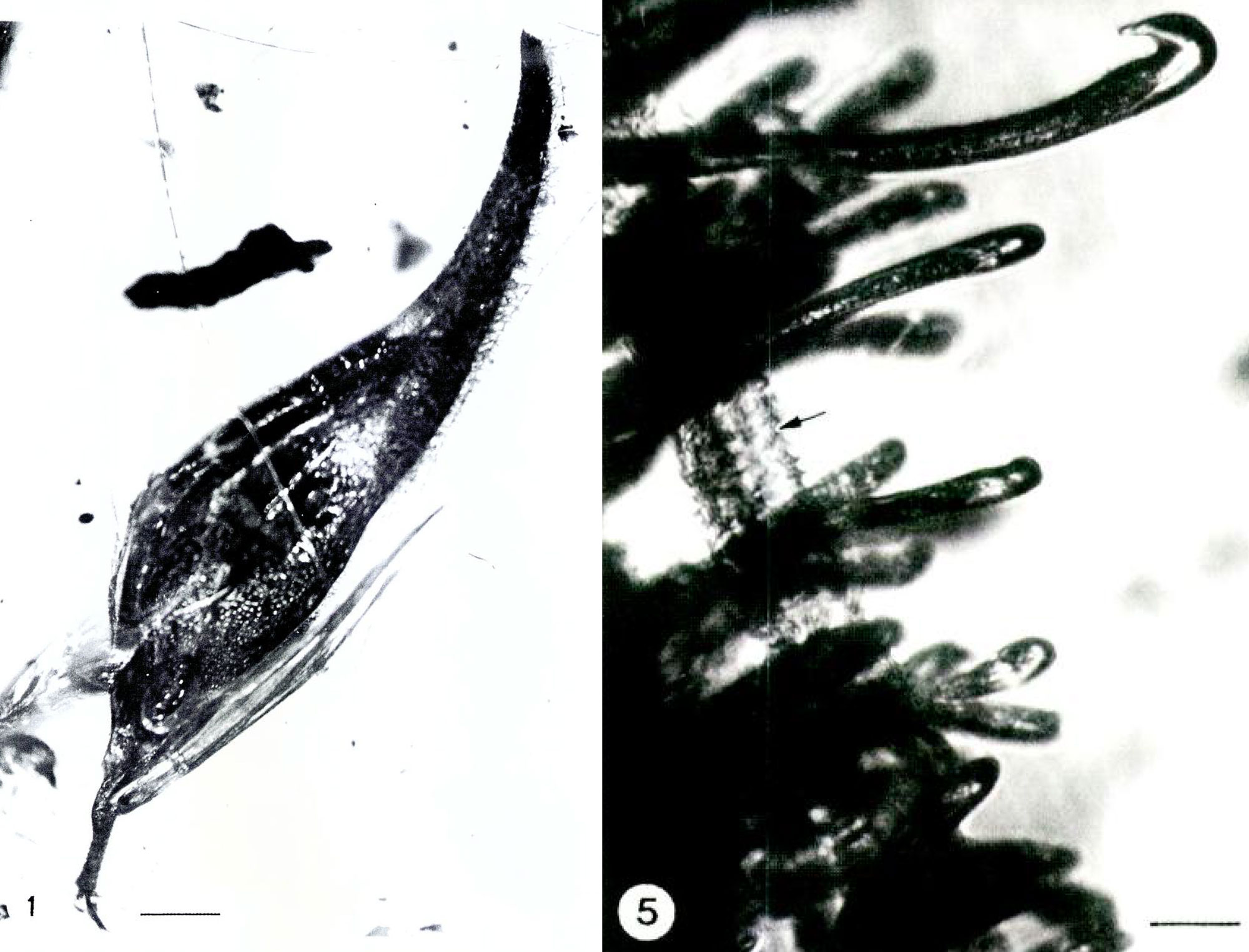
Pharus primuncinatus from Miocene Dominican amber. Left: The spikelet, scale bar = 0.86 mm. Right: Detail of hooked hairs on the outside of the spikelet; the arrow points to a mammal hair. Scale bar = 58 microns. Source: Figures 1 and 2 from Poinar & Judziewicz (2005) SIDA (Biodiversity Heritage Library, Creative Commons-NonCommercial-ShareAlike 3.0 Unported license, images cropped and reconfigured).
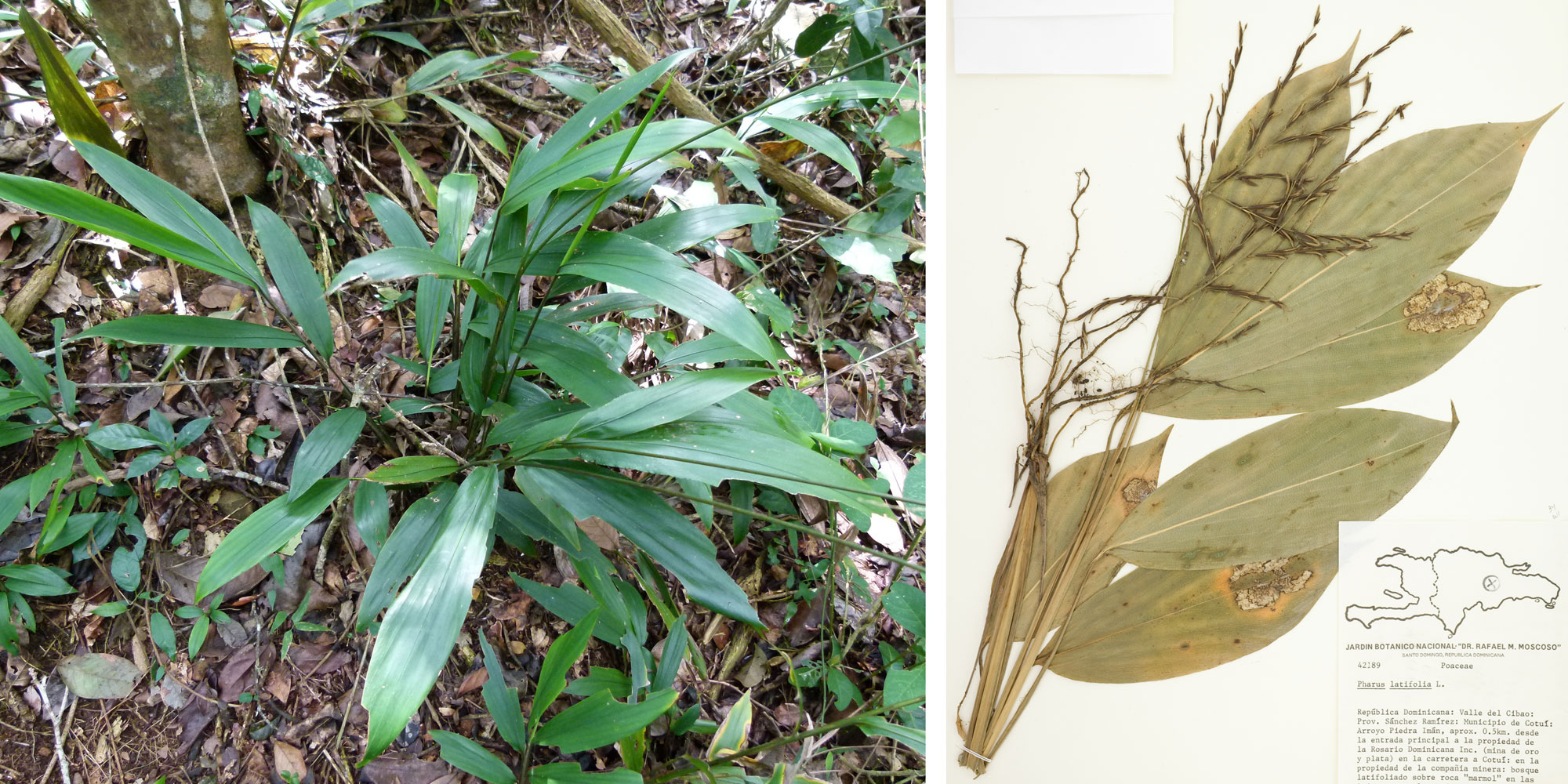
Examples of modern stalkgrasses (Pharus, subfamily Pharoideae). Left: Creeping leafstalk grass (Pharus lappulaceus), Cuyón, Aibonito, Puerto Rico. Right: Herbarium specimen of Pharus latifolius from the Dominican Republic. Left photo by botanygirl (iNaturalist, Creative Commons Attribution 4.0 International license, image cropped and resized). Right photo of NY1606841 by New York Botanical Garden (NYBG on GBIF.org, Creative Commons Attribution 4.0 International license, image cropped and resized).
The earliest origins of C4 photosynthesis are estimated to date back to the Oligocene based on molecular dating techiques. Stable isotope analysis of fossil grass pollen also supports the appearance by C4 grasses by the Oligocene. The earliest evidence for C4 grass-dominated habitats is from the early Miocene of Africa (discussed above).
The earliest known C4 grass macrofossils, however, are from the late Miocene. One comes from the Miocene Dove Spring Formation, California, U.S.A., and is about 12.5 to 8 million years old; the other comes from the Miocene Ogallala Formation of Kansas, U.S.A., and is about 7 to 5 million years old. These fossil grasses have anatomical (cellular) preservation that shows the characteristic Kranz anatomy of C4 grass leaves. Kranz, meaning wreath, refers to the concentric arrangement of the mesophyll cells around the vascular bundles in C4 grasses when they are viewed in cross section.
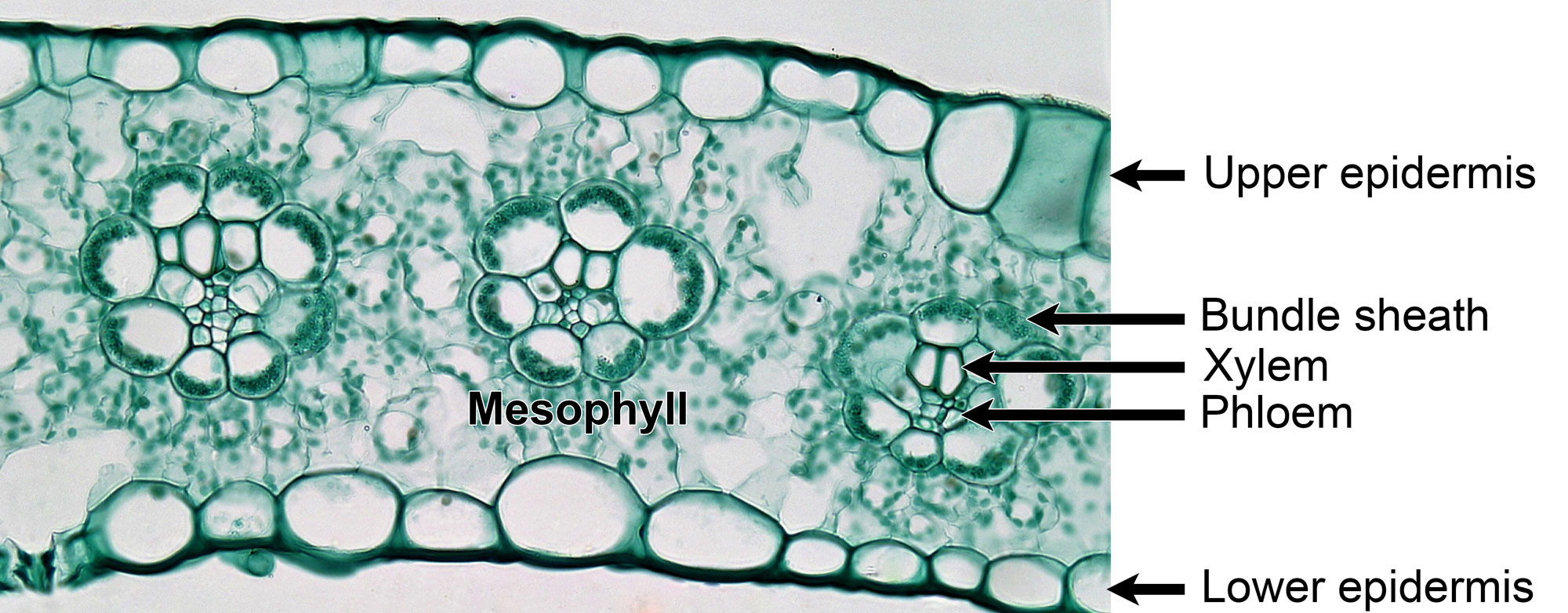
Fixed and stained cross section of a leaf of maize (corn, Zea mays), an extant C4 grass, showing three veins, each with vascular tissue surrounded by a bundle sheath. Mesophyll cells encircle the bundle sheaths, making up the characteristic Kranz anatomy of C4 grasses. Photo by Berkshire Community College (flickr, CC0 1.0 Univerisal/public domain dedication).
Pleistocene to recent grasslands (2.6 million years ago to present)
During the Pleistocene, polar ice caps formed in the Northern Hemisphere and spread southward multiple times, alternately covering and exposing the northern parts of North America and well as some parts of northern Eurasia. As today, an ice sheet also covered Antarctica. Alpine glaciers were more extensive than they are today. Large parts of the globe were covered by grasslands, including tropical grassland savanna and steppes.
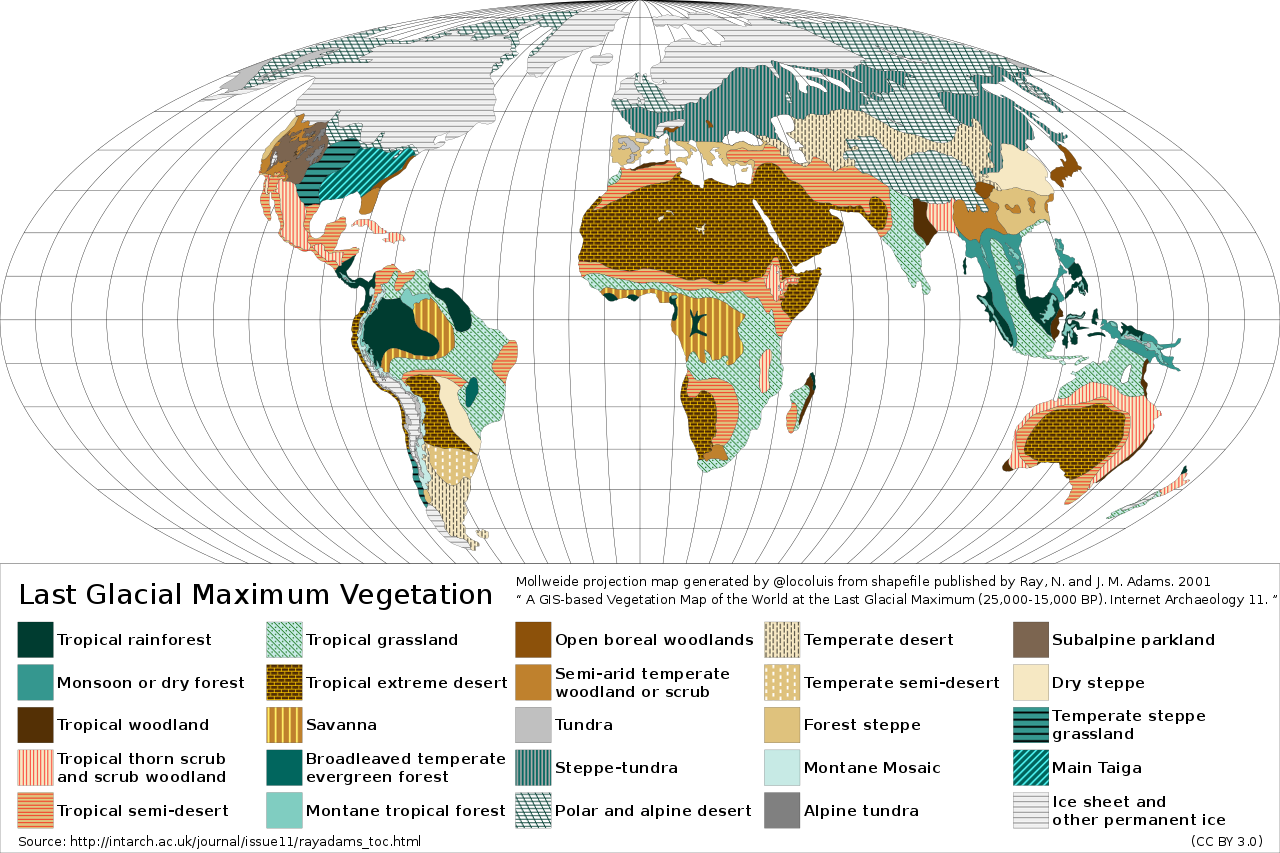
A map of vegetation during the last glacial maximum. Photo by Locoluis, generated from shapefile published by Ray, N. and J. M. Adams. 2001, “ A GIS-based Vegetation Map of the World at the Last Glacial Maximum (25,000-15,000 BP). Internet Archaeology 11. ” Licensed under a Creative Commons Attribution 3.0 Unported license.
A unique feature of the Pleistocene is the mammoth-steppe or steppe-tundra biome, which was, at the time of the last glacial maximum (about 26,000 to 19,000 years ago), the world’s most extensive biome. The mammoth-steppe was a grass-dominated ecosystem. Various lines of evidence, including macrofossils, phytoliths, and stable isotopes, show an abundance of graminoids (grasses and grass-like plants) in this biome. Additionally, grass DNA preserved in permafrost has provided evidence of specific species growing in high northern latitudes during the Pleistocene.
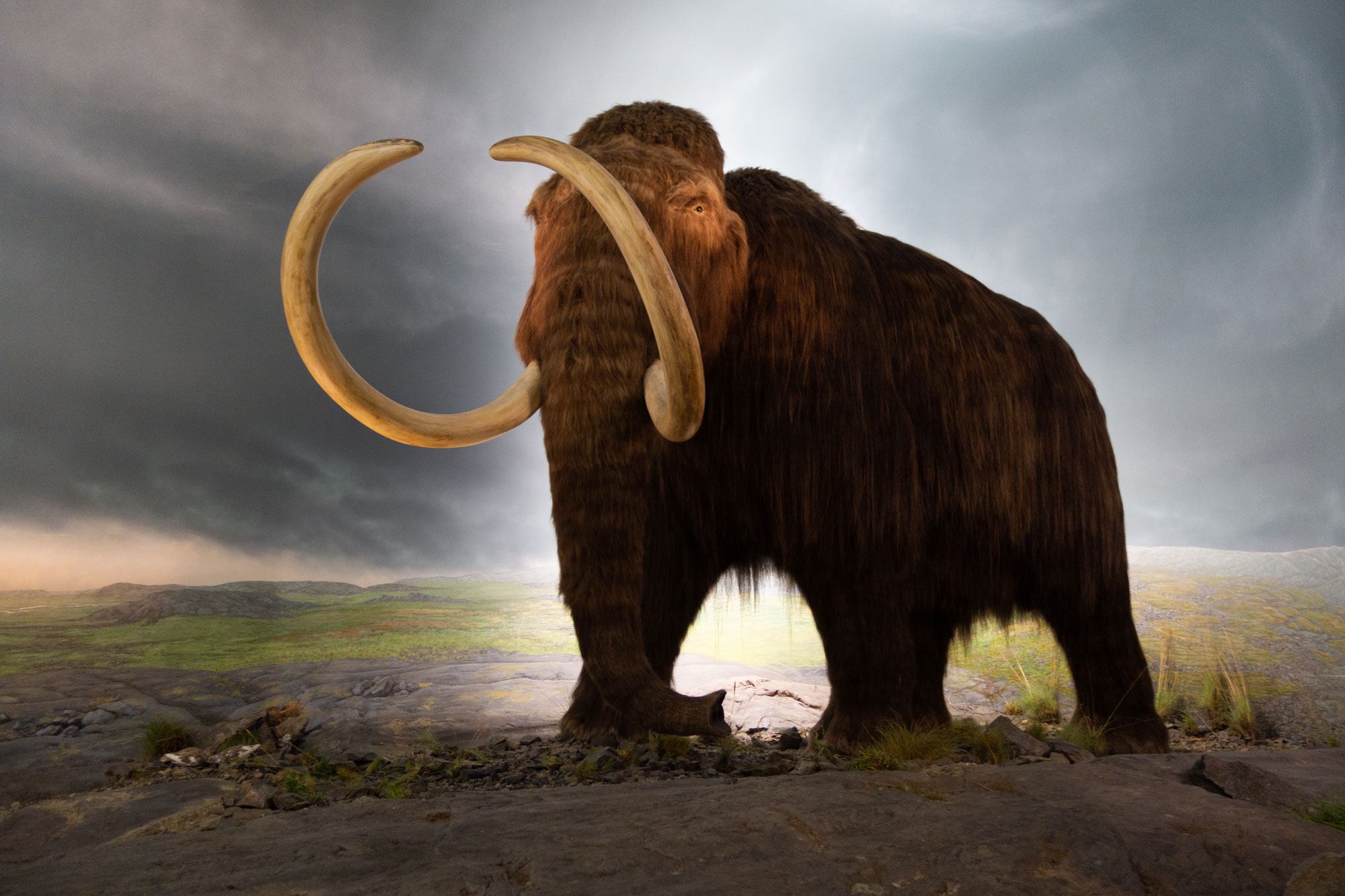
Model of a woolly mammoth on display at the Royal Victoria Museum, British Columbia, Canada. Photo by Thomas Quine (Wikimedia Commons, Creative Commons Attribution 2.0 Generic license, image resized).
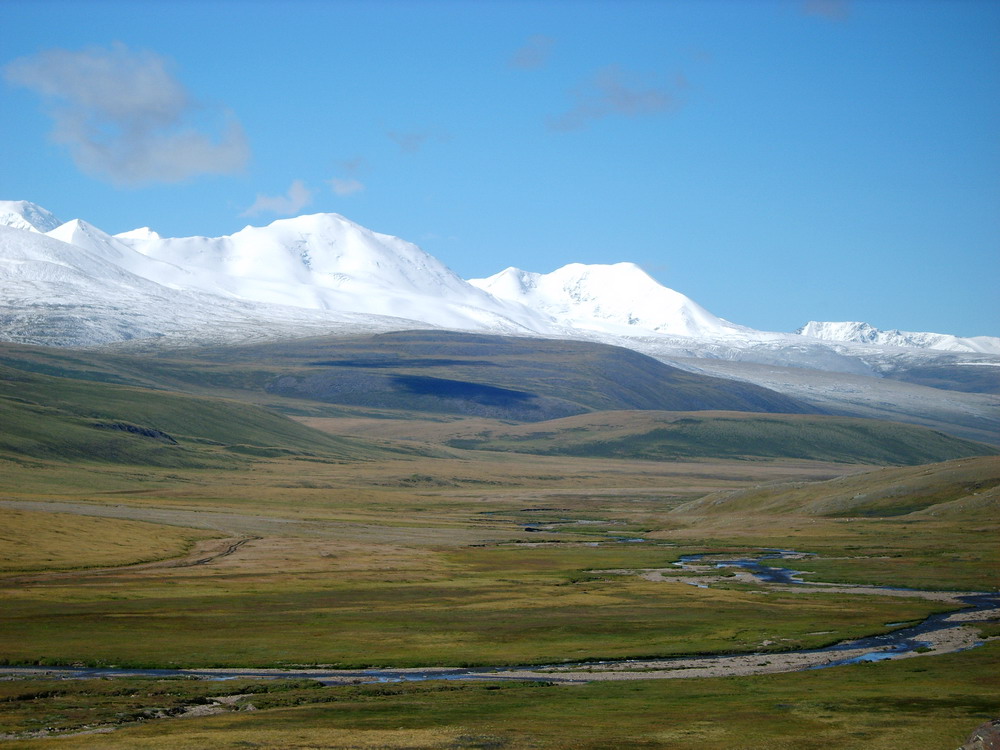
The Ukok Plateau in Siberia, Russia. The Altai Mountains region, where the Ukok Plateau is located, is similar to (and may be a remnant of) the once widespread Pleistocene mammoth-steppe (steppe-tundra) grassland biome. Photo by Kobsev (Wikimedia Commons, Creative Commons Attribution 2.5 Generic license).
As the Earth warmed, the glaciers receded following the last glacial maximum, and land that was formerly covered by ice was exposed. Eventually, grasslands and savannas assumed their present distributions. Near the end of the Pleistocene to the early Holocene, around 10,000 years ago or even earlier, humans began to cultivate and domesticate grasses. In the modern world, many grasslands have been severely disturbed or completely replaced by fields of cultivated plants, livestock grazing, and development.
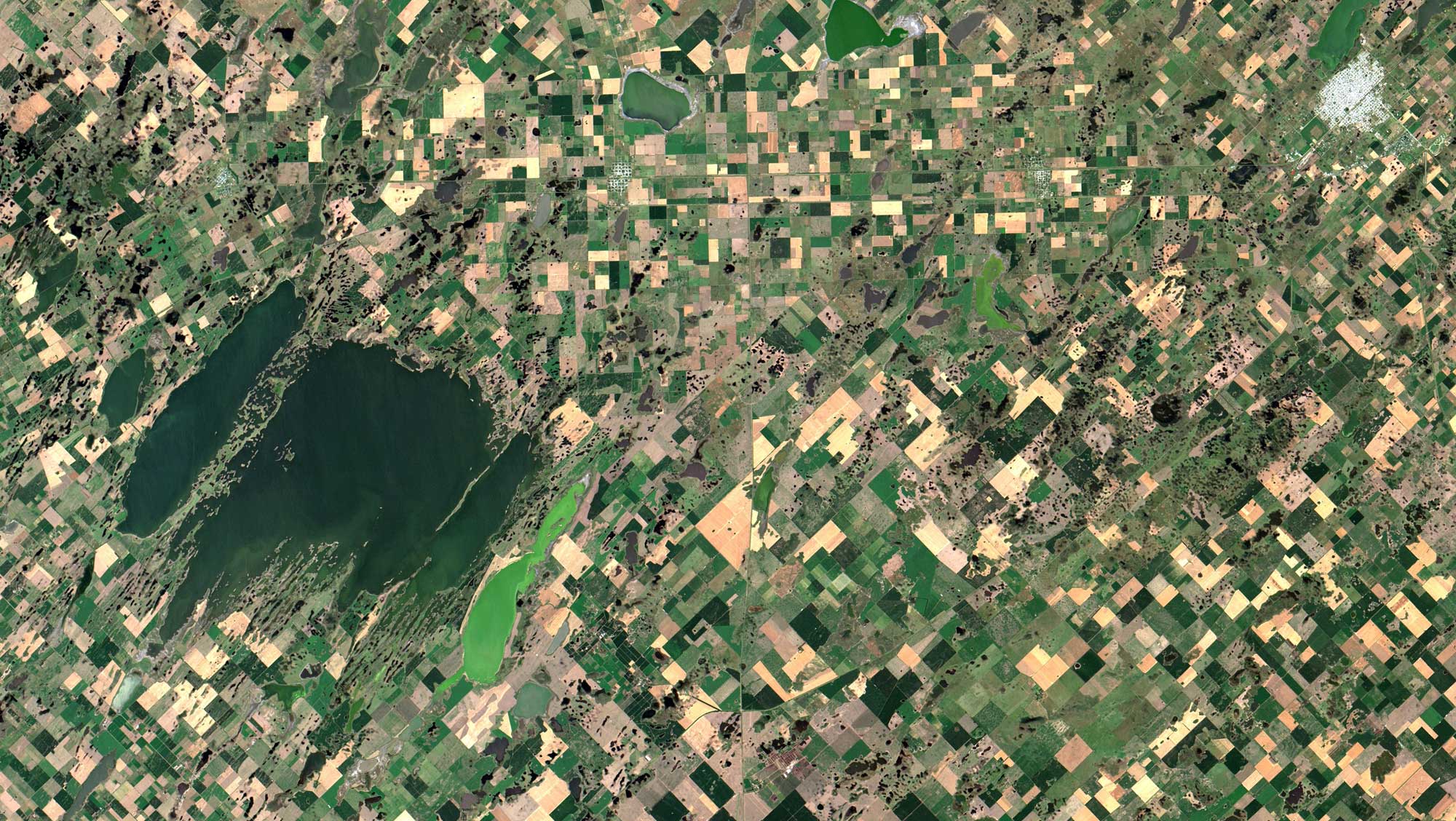
Satellite view of the humid pampas region of Córdoba, Argentina, showing the Las Tunas lake system, 2018. Note that much of this grassland region has been altered by agriculture, as have many grasslands that occur in climates that can support intensive farming. Photo by Oton Barros DSR/OBT/INPE (Coordenação-Geral de Observação da Terra/INPE, Creative Commons Attribution-ShareAlike 2.0 Generic license).
Resources
Websites
Angiosperm Phylogeny Website (P. F. Stevens): http://www.mobot.org/MOBOT/research/APweb/
GrassWorld: https://grassworld.myspecies.info/en
Variations in photorespiration (Photosynthesis: It brings good things to life, University of California Museum of Paleontology): https://ucmp.berkeley.edu/glossary/gloss3/photosyn/respiration.html
Articles
Cutts, E. 2021. Mammoths lost their steppe habitat to climate change. Eos, 19 November 2021. https://eos.org/articles/mammoths-lost-their-steppe-habitat-to-climate-change
Scientific articles and books
Crepet, W. L., and G. D. Feldman. 1991. The earliest remains of grasses in the fossil record. American Journal of Botany 78: 1010-1014. https://doi.org/10.2307/2445181
Gallaher, T. J., P. M. Peterson, R. J. Soreng, F. O. Zuloaga, D. Li, L. G. Clark, C. D. Tyrrell, C. A. Welker, E. A. Kellogg, and J. K. Teisher. 2022. Grasses through space and time: An overview of the biogeographical and macroevolutionary history of Poaceae. Journal of Systematics and Evolution 60: 522-569. https://doi.org/10.1111/jse.12857
Jacobs, B. F., J. D. Kingston, and L. L. Jacobs. 1999. The origin of grass-dominated ecosystems. Annals of the Missouri Botanical Garden 86: 590-643. https://doi.org/10.2307/2666186
Kellogg, E. A. 2015. Flowering Plants, Monocots, Poaceae. The Families and Genera of Vascular Plants 13, 416 pp. (K. Kubitzki, ed.). Springer International Publishing, Switzerland. https://doi.org/10.1007/978-3-319-15332-2_1
Kukla, T., J. K. C. Rugenstein, D. E. Ibarra, M. J. Winnick, C. A. E. Strömberg, and C. P. Chamberlain. 2022. Drier winters drove Cenozoic open habitat expansion in North America. AGU Advances 3: e2021AV000566. https://doi.org/10.1029/2021AV000566
Manchester, S. R. 2001. Update on the megafossil flora of Florissant, Colorado. Denver Museum of Nature & Science, Series 4(1): 137-161.
Meyer, H. W. 2003. The fossils of Florissant. Smithsonian Books, Washington.
Nambudiri, E. M. V., W. D. Tidwell, B. N. Smith, and N. P. Hebbert. 1978. A C4 plant from the Pliocene. Nature 276: 816-817.
Peppe, D. J., S. M. Cote, A. L. Deino, D. L. Fox, J. D. Kingston, R. N. Kinyanjui, W. E. Lukens, L. M. Maclatchy, A. Novello, C. E. Strömberg, S. G. Driese, N. D. Garrett, K. R. Hillis, B. F. Jacobs, K. E. H. Jenkins, R. M. Kityo, T. Lehmann, F. K. Manthi, E. N. Mbua, L. A. Michel, E. R. Miller, A. A. T. Mugume, S. N. Muteti, I. O. Nengo, K. O. Oginga, S. R. Phelps, P. Polissar, J. B. Rossie, N. J. Stevens, K. T. Uno, and K. P. McNulty. 2023. Oldest evidence of abundant C4 grasses and habitat heterogeneity in eastern Africa. Science 380: 173-177. https://doi.org/10.1126/science.abq2834
Poinar, G. O., Jr. 2004. Programinis burmitis gen. et. sp. nov., and P. laminatus sp. nov., Early Cretaceous grass-like monocots in Burmese amber. Australian Systematic Botany 17: 497-504.
Poinar, G. O., Jr. 2011. Silica bodies in Early Cretaceous Programinis laminatus (Angiospermae: Poales). Palaeodiversity 4: 1-6.
Poinar, G. O., Jr., and J. T. Columbus. 1992. Adhesive grass spikelet with mammalian hair in Dominican amber: First fossil evidence of epizoochory. Experientia 48: 906-908. https://doi.org/10.1007/BF02118433
Poinar, G., Jr., and J. T. Columbus. 2012. Alarista succina gen. et sp. nov. (Poaceae: Bambusoideae) in Dominican amber. Historical Biology 25: 691-696. https://doi.org/10.1080/08912963.2012.742891
Poinar, G., and E. J. Judziewicz. 2005. Pharus primuncinatus (Poaceae: Pharoideae: Phareae) from Dominican amber. SIDA, Contributions to Botany 21: 2095-2103.
Poinar, G., Jr., and R. J. Soreng. 2021. A new genus and species of grass, Eograminis balticus (Poaceae: Arundinoideae), in Baltic amber. International Journal of Plant Sciences 182: 808-816. https://doi.org/10.1086/716781
Poinar, G., Jr., S. Alderman, and J. Wunderlich. 2015. One hundred million year old ergot: psychotropic compounds in the Cretaceous. Paleodiversity8: 13-19.
Prasad, V., C. A. E. Strömberg, H. Alimohammadian, and A. Sahni. 2005. Dinosaur coprolites and the early evolution of grasses and grazers. Science 310: 1177-1180. https://www.science.org/doi/10.1126/science.1118806
Prasad, V., C. A. E. Strömberg, A. D. Leaché, B. Samant, R. Patnaik, L. Tang, D. M. Mohabey, S. Ge. and A. Sahni. 2011. Late Cretaceous origin of the rice tribe provides evidence for early diversification in Poaceae. Nature Communications 2: 480. https://doi.org/10.1038/ncomms1482
Sadowski, E.-M., A. R. Schmidt, P. J. Rudall, D. A. Simpson, C. Gröhn, J. Wunderlich, L. J. Seyfullah. 2016. Graminids from Eocene Baltic amber. Review of Palaeobotany and Palynology 233: 161-168. https://doi.org/10.1016/j.revpalbo.2016.02.005
Strömberg, C. A. E. 2011. Evolution of grasses and grassland ecosystems. Annual Review of Earth and Planetary Sciences 39: 517-544. https://doi.org/10.1146/annurev-earth-040809-152402
Strömberg, C. A. E., and A. C. Staver. 2022. The history and challenge of grassy biomes. Science 377: 6606. https://doi.org/10.1126/science.add1347
Thomasson, J. R., M. E. Nelson, and R. J. Zakrzewski. 1986. A fossil grass (Gramineae: Chloridoideae) from the Miocene with Kranz anatomy. Science 233: 876-878.
Urban, M. A., D. M. Nelson, G. Jiménez-Moreno, J.-J. Châteauneuff, A. Pearson, and F. S. Hu. 2010. Isotopic evidence of C4 grasses in southwestern Europe during the early Oligocene-middle Miocene. Geology 38: 1091-1094. https://doi.org/10.1130/G31117.1
Wilf, P. 2020. Eocene "Chusquea" fossil from Patagonia is a conifer, not a bamboo. PhytoKeys 139: 77-89. https://doi.org/10.3897/phytokeys.139.48717
Wu, Y., H.-L. You, and X.-Q. Li. 2018. Dinosaur-associated Poaceae epidermis and phytoliths from the Early Cretaceous of China. National Science Review 5: 721-727. https://doi.org/10.1093/nsr/nwx145