Page snapshot: How is a changing climate affecting agricultural grasses, and how are growers adapting? How will climate change affect wild grasslands? Can grasslands be part of climate change solutions? This page addresses these questions.
Topics covered on this page: Introduction; Impacts of Climate Change on Grasses; Grasslands as Climate Change Solutions; References; Resources
Credits: Funded by the National Science Foundation. Any opinions, findings, and conclusions or recommendations expressed in this material are those of the author(s) and do not necessarily reflect the views of the National Science Foundation. Page by Ingrid H. H. Zabel (2023)
Updates: Page last updated August 31, 2023.
Image above: A farmer in Clay County, Texas, in his field where he has adopted no-till farming and the use of cover crops to increase his wheat crop's drought hardiness. Photo by USDA NRCS Texas (CC BY 2.0 license) via Flickr.
Introduction
It’s hard to think of a major sector of human activity more closely tied to climate and weather than agriculture. For example, most of the wheat in the world grows in North America, Europe, and Asia because of the temperature climate of these regions. We rely on grass crops such as wheat, maize, rice, and sugarcane, so their response to a changing climate and our ability to continue to grow them successfully are of great concern.
Humans developed agriculture over about the last 10,000 years, during a time of relatively stable global climate compared with the previous 10,000 years. The figure below shows that within the last 10,000 years, the Earth’s average surface temperature has not varied more than about ±1°C, whereas between 20,000 and 10,000 years ago, the Earth’s average temperature changed by almost 7°C.
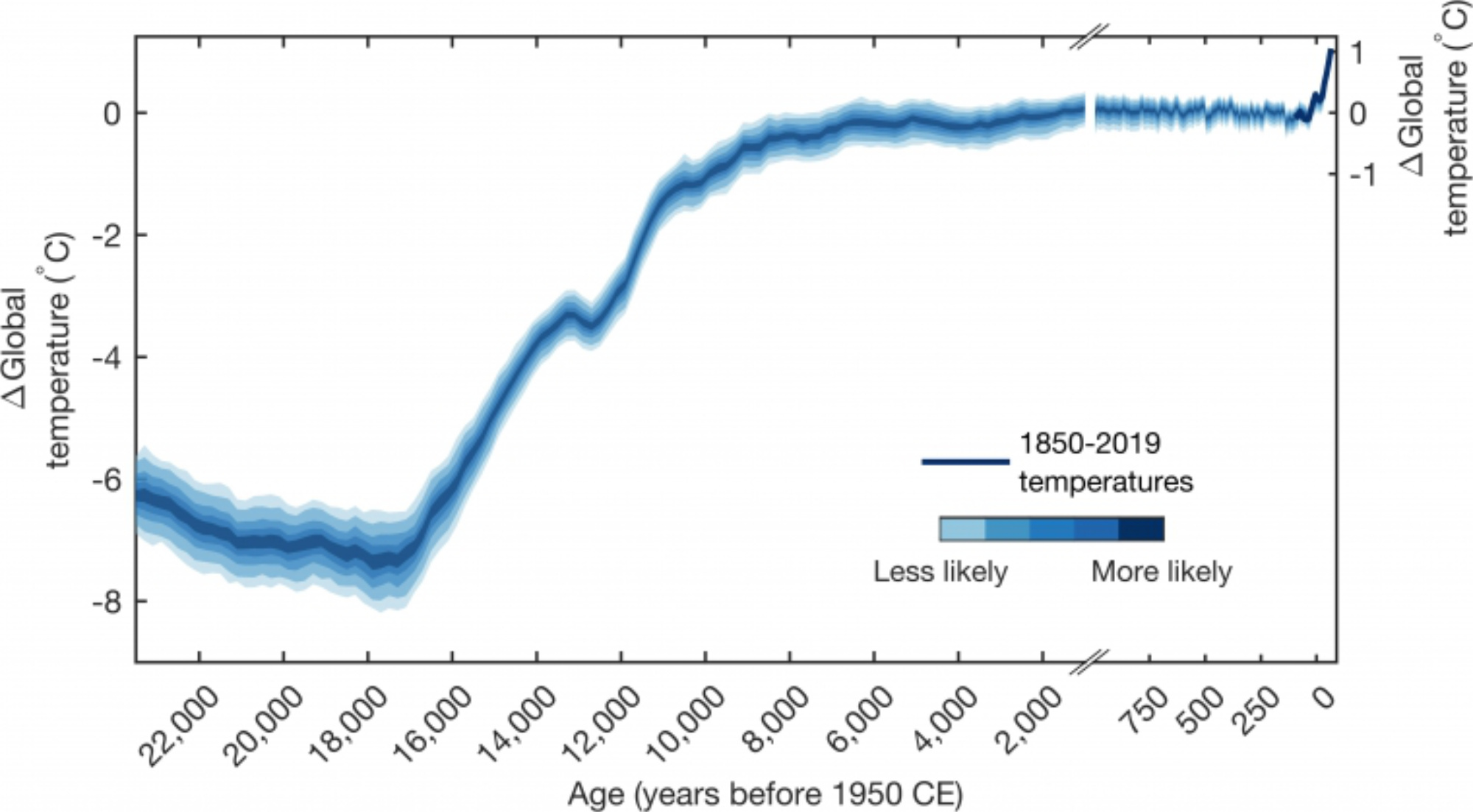
Reconstruction of global average surface temperature anomaly. Temperature anomaly is the change in temperature relative to some baseline; in this case, the baseline is the preindustrial last millennium average, i.e., average temperature from 1000–1850 CE. Graph by Matthew Osman, from Global Temperatures Over Last 24,000 Years Show Today's Warming 'Unprecedented' by Mikayla Mace Kelley, U. of Arizona University Communications, Nov. 10, 2021 (accessed July 26, 2023). The data in this graph come from the study Osman, M. B. et al. Globally resolved surface temperatures since the Last Glacial Maximum. Nature 599, 239–244 (2021). https://www.nature.com/articles/s41586-021-03984-4
Today we are polluting the atmosphere with greenhouse gases that are heating the planet rapidly, at a rate unprecedented in geologic history. This heating leads to changes in precipitation patterns and storms—the other parts of climate change in addition to temperature change. These changes can have destructive consequences such as drought, flooding, sea level rise, ecosystem changes, and increasing health risks for people, animals, and plants.
Impacts of Climate Change on Grasses
Agricultural Grasses
Climate change can affect crop growth in a wide variety of ways: warmer air can dry out the soil through evaporation, and crops can experience heat stress during heat waves. Increases in rainfall or decreases in runoff of snowmelt can impact crop growth. The response of weeds and insect pests to a changing climate affects crops as well. These impacts, and more, are summarized in the table below (adapted from Responding to Climate Change in New York State (ClimAID), Chapter 12, 2011). [1]
Climate Variable
Impact
Consequence Without Adaptation
Increase in average temperature
Longer growing season for certain crops
Potentially increased crop yield and expanded market opportunity for some crops, along with potential price drops. Increased seasonal water and nutrient requirements.
Increased weed, disease, and insect pressure
Lower native crop survival; increases in prices
Weed species more resistant to herbicides
Change in species composition potentially not favoring native crops
Northward expansion of disease range and weeds
Lower crop survival
More insect pest generations per season; shifts in species ranges
Increased vulnerability of crops to pests
Warmer winters
More winter cover crop options
Increased spring populations of marginally overwintering insects; northward range expansion of invasive weeds
Increased vulnerability of crops to pests and invasives
Increase in extreme heat events
Crop stress, especially if extreme events occur in clusters
Major crop and profit loss
Heat stress effects
Negatively affect yield or quality of cool-season crops
Increase in average precipitation
Increased flooding, resulting in inability to access fields during critical times, and possible delay of spring planting and harvest.
Direct crop damage, increased chemical contamination of waterways and harvested crops, negative effect on market prices
Increased soil compaction because of tractor use on wet soils
Increased vulnerability to future flooding and drought; increasing runoff and erosion; difficulty for plant root growth
Increased crop root disease and anoxia
Decrease in crop productivity and yield
Wash-off of applied chemicals
Decrease in crop productivity and yield
Increase in heavy rainfalls
Crop stress, especially if events occur in clusters
Major crop and profit loss
Increase in drought
Increased plant stress
Reduced yields and crops losses, especially for rain-fed agriculture
Inadequate irrigation capacity for some growers
Decrease in crop yield
Dry streams or wells
Increased pumping cost from wells
Table contents from Table 12.2 of Chapter 12: Conclusions and Recommendations in Rosenzweig, C., W. Solecki, A. DeGaetano, M. O'Grady, S. Hassol, P. Grabhorn (Eds.). 2011. Responding to Climate Change in New York State: The ClimAID Integrated Assessment for Effective Climate Change Adaptation.Technical Report (accessed August 9, 2023). New York State Energy Research and Development Authority (NYSERDA), Albany, New York.
The Intergovernmental Panel on Climate Change (IPCC) has found that climate change has already affected grass crops in a variety of ways, with growing regions expanding toward the poles as the planet warms, cold stress declining in North America and East Asia, and crop yields increasing in some parts of the world and decreasing in others. According to the IPCC's 2022 report,
"Climate impacts for the past 20–50 years differ by crops and regions. Positive effects have been identified for rice and wheat in Eastern Asia, and for wheat in Northern Europe. The effects are mostly negative in Sub-Saharan Africa, South America and Caribbean, Southern Asia, and Western and Southern Europe." [2]
The IPCC also concluded that extreme weather events such as drought, heat waves, and flooding are having increasingly damaging effects on grass crops. For example, from 1961 to 2014, drought and extreme heat combined have reduced global average yields of wheat by 9.2% and maize by 11.6%. [3]
As for future climate change, the IPCC reports that "climate change will disproportionately affect crop yields among regions, with more negative than positive effects being expected in most areas, especially in currently warm regions, including Africa and Central and South America (high confidence)."
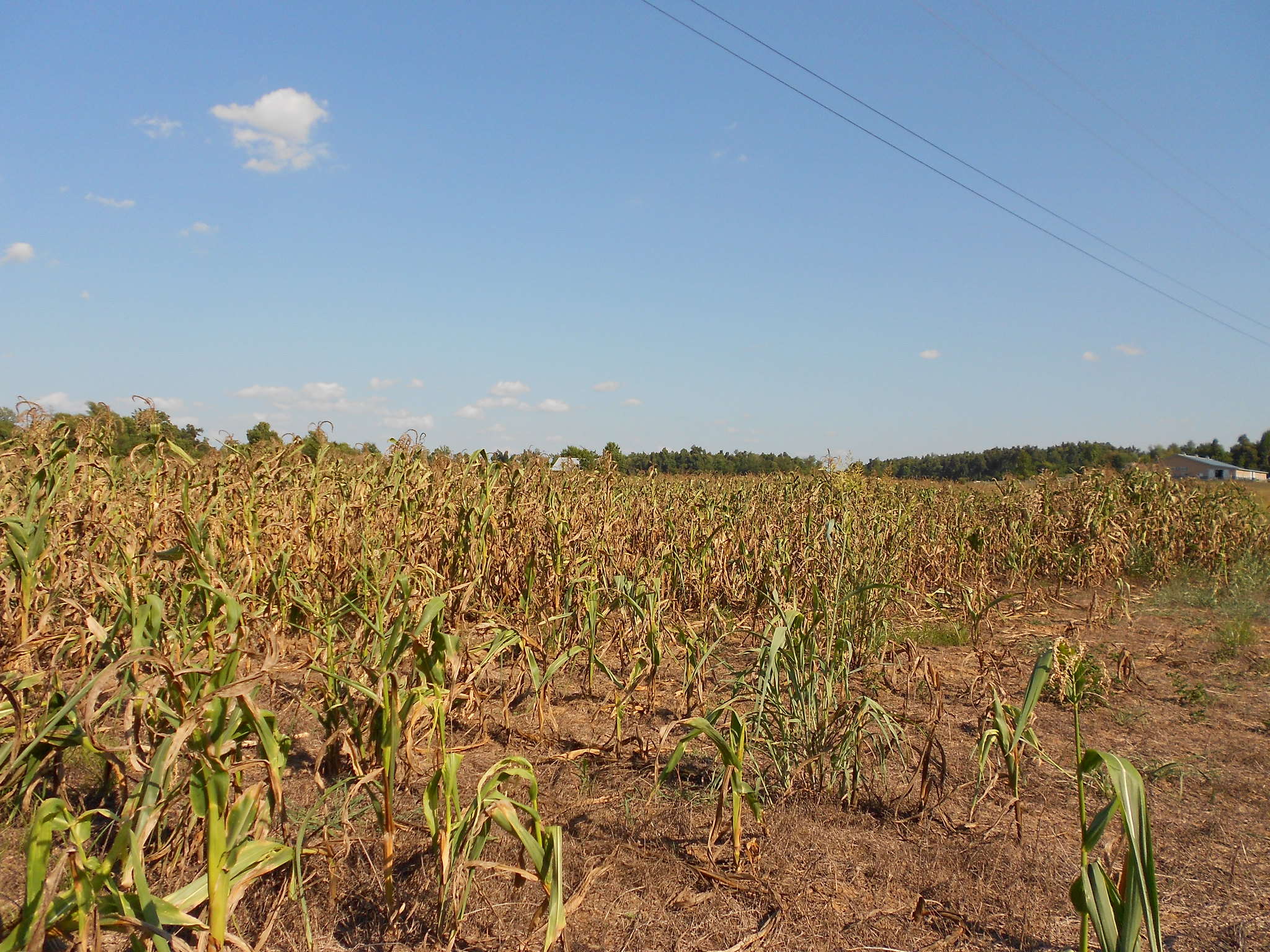
Drought-stressed corn. Photo by CraneStation (CC BY 2.0 license) via Flickr
In addition to tracking the effects of climate change on grass crops, scientists have been studying how increased carbon dioxide (CO₂) concentration in the atmosphere is affecting plant growth. Scientists have used a technique called Free-Air Carbon Dioxide Enrichment (FACE) to grow crops in open air over multiple growing seasons, with equipment to raise the CO₂ level of the air. Experiments show that higher CO₂ concentrations lead to increased photosynthesis and enhanced growth of C₃ crops (see Glossary for definition of a C₃ plant.) However, the IPCC cites multiple studies that show "positive effects of rising CO₂ on yield are significantly reduced by higher temperatures" [2] for both wheat and rice.
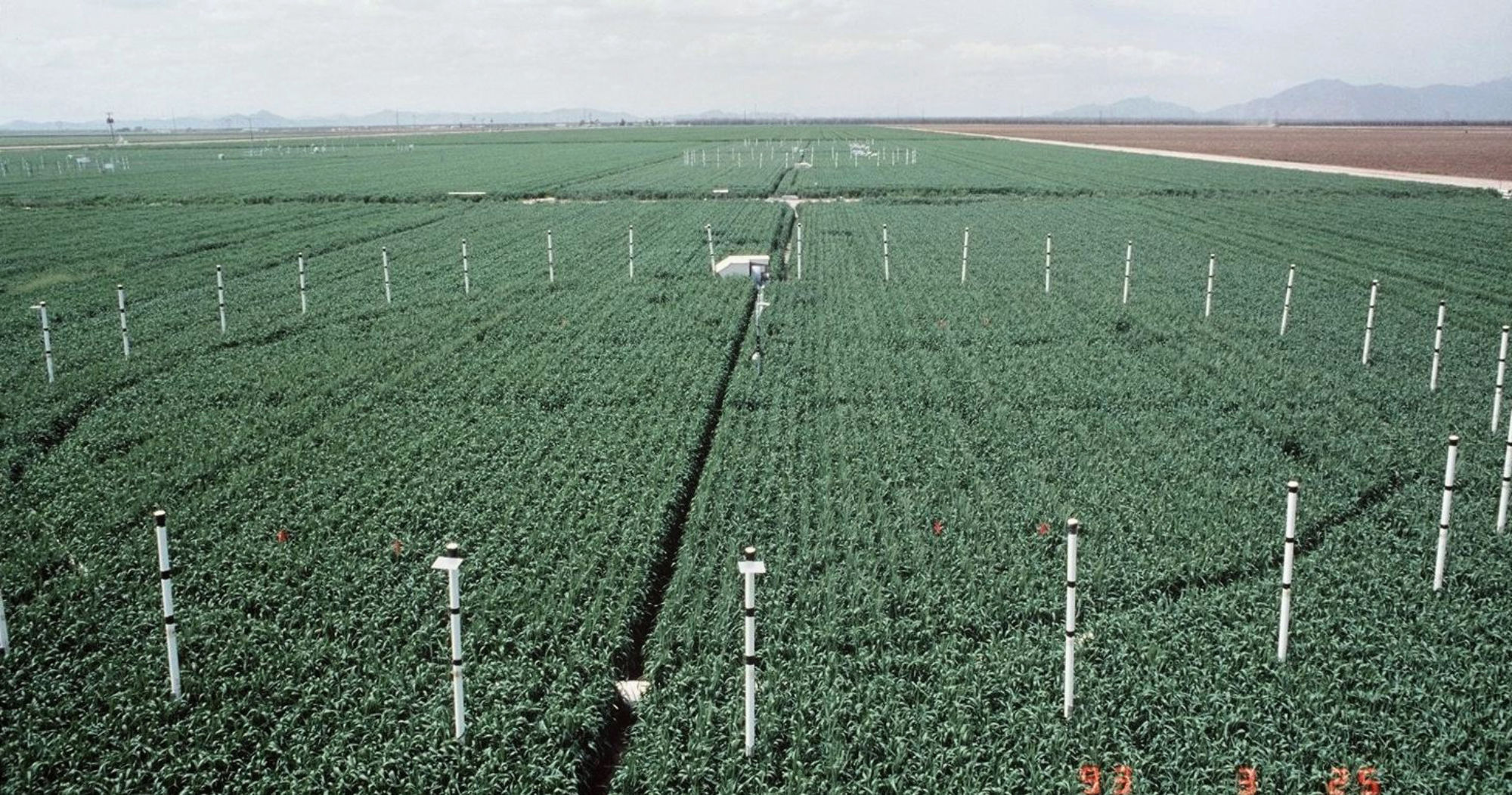
Agricultural Adaptation
Agricultural researchers and farmers are developing and implementing a variety of strategies to adapt to a changing climate. These include:
- Developing new cultivars (selectively bred varieties) that can better withstand drought and/or heat, or that mature at different speeds than in the past
- Shifting planting and harvesting times to respond to changing growing seasons
- Using new ways of managing pests, weeds, and diseases
- Using irrigation and flood management systems
- Optimizing soil health by practicing conservation agriculture, such as disturbing the soil as little as possible, rotating crops, and adding carbon to the soil.
- Reducing risk by growing different crops together, such as wheat and barley. If one crop fails due to climate hazards, the other may still survive.
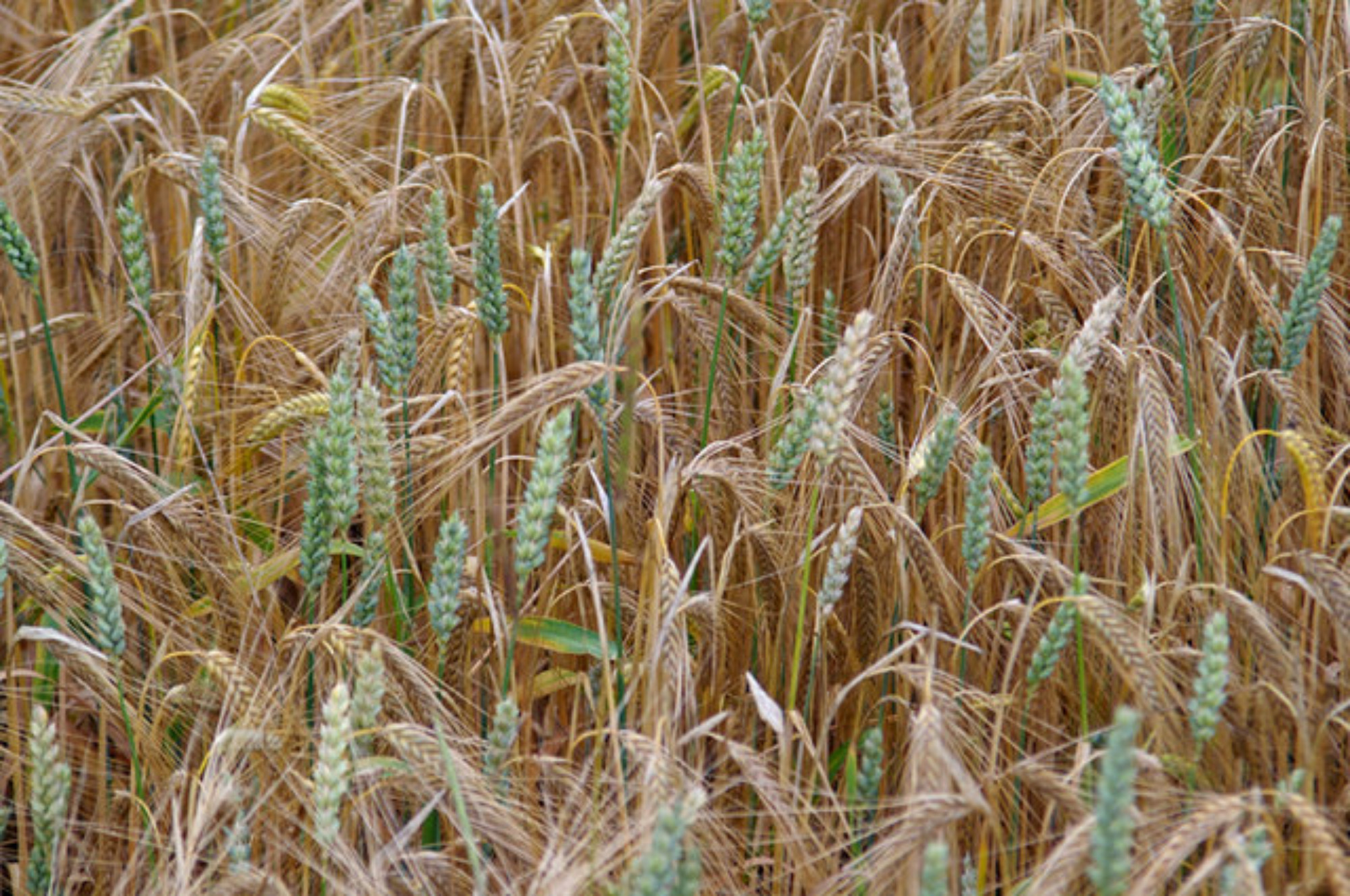
Wild Grasslands
Wild grasslands experience the effects of climate change just as agricultural grasslands do, though the outcomes may be different as they are managed (or left unmanaged) differently. The fate of semi-wild grasslands that are used for grazing has implications for livestock agriculture. Wild grasslands provide rich habitat for wildlife, and the impacts of climate change on grasslands can have implications for biodiversity.
In western North America, models project that by the end of the 21st century, rising temperatures and lack of water will lead to about half of evergreen tree cover being replaced by shrubs and grasses. [4] Other projected grassland range shifts in the U.S. [5] are summarized as follows by the USDA Forest Service [6]:
- "Climate suitable for Great Plains grasslands is expected to remain relatively stable with some expansion to the north in Canada and retraction on the eastern and southern boundaries.
- Climate suitable for semi desert grasslands in the southwestern U.S. is expected to expand while contracting in Mexico.
- Climate suitable for Great Basin shrub-grasslands is expected to decline with contraction primarily along the eastern boundary in Colorado, Montana, and eastern Idaho.
- Climate suitable for California valley grasslands is likely to have significant declines, shifting towards oak woodlands and desert scrub, along with a high proportion of no analog climates (i.e., projected climates do not match any contemporary biomes).
- Climate suitable for Gulf Coastal grasslands is expected to contract towards southeastern Texas and have a high proportion of no analog climates."
"Other expected changes not captured by the distribution models include effects of CO2 and nitrogen availability."
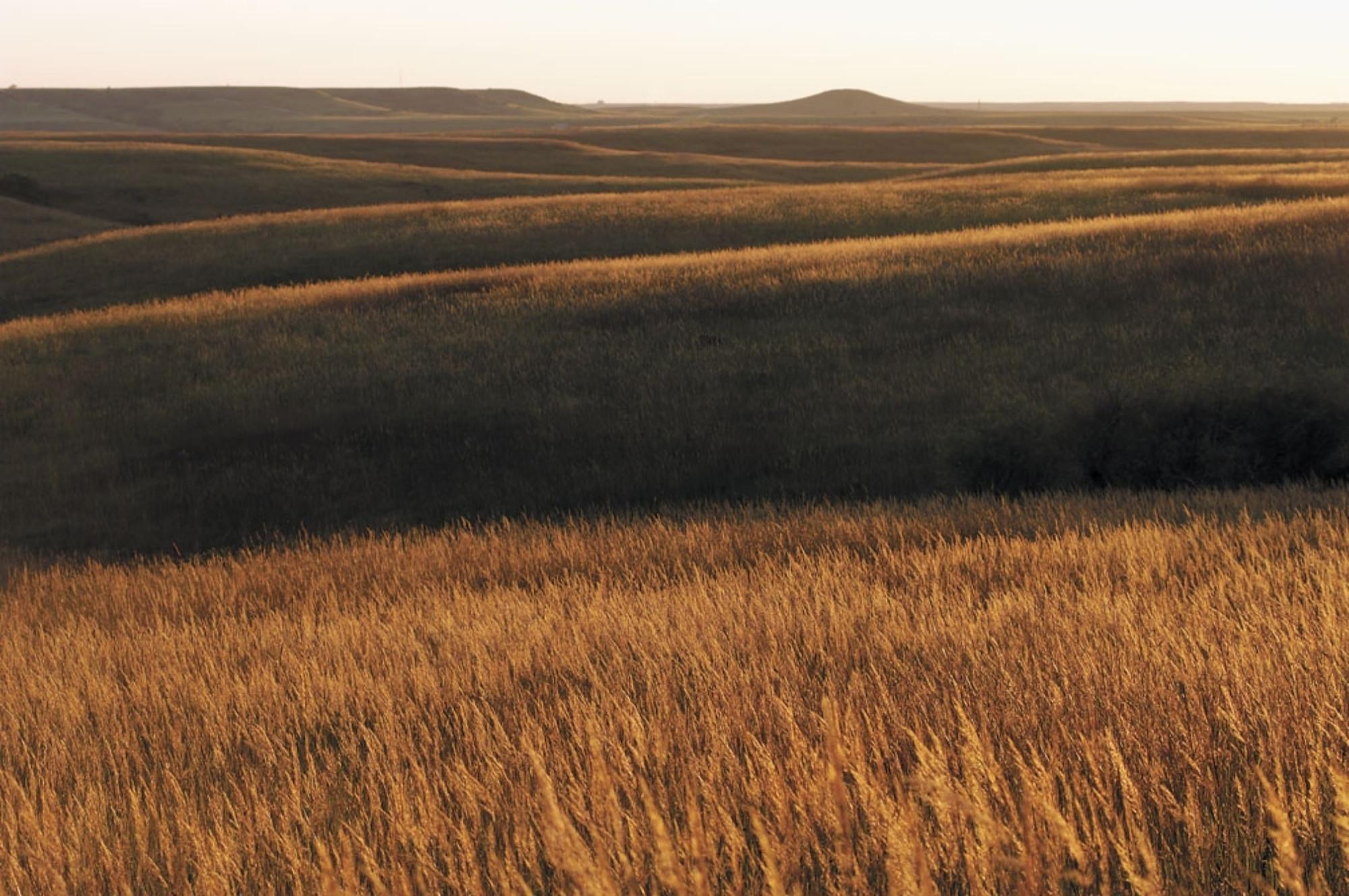
Heavy precipitation events are expected to increase in many parts of the world as the climate warms. An experiment on grasses and woody plants that were subjected to heavy precipitation showed that it can result in water reaching deeper in the soil, leading to increased growth of woody plants which crowded out grasses. [7] This could have implications for woody plants moving into grasslands in the future.
Increasing atmospheric CO₂ levels affects natural grasslands as well as agricultural crops. A study of a mixed-grass prairie in Wyoming found that while increasing CO₂ increased plant productivity, the digestibility and protein content of one of the grasses, western wheatgrass, declined, which would be detrimental for cattle grazing. [8]
Another study showed different responses of C₃ and C₄ grasses to climate change. C₃ grasses have thrived during cool seasons and in cooler regions, and C₄ grasses have thrived in warmer regions with more warm-season rain and more temperature variability. Models which incorporated future climate scenarios showed that "C3 grass abundance declined across 74% of areas, while C4 abundance increased across 66% of areas. C3 grasses expanded in mid- to higher-latitude areas with increasing temperature and decreasing seasonality of precipitation. In contrast, C4 grasses increased in higher-latitude regions, but declined in lower-latitude, dryer regions." [9]
Grasslands as Climate Change Solutions
Grassland Carbon Sequestration: Capturing and Storing Carbon
In 2018, the IPCC concluded that every pathway to limiting global heating to 1.5°C above pre-industrial temperature, with limited or no overshoot, would involve removing CO₂ from the atmosphere. [10] Plants remove CO₂ from the air through photosynthesis, and they store it in their tissues and move it to the soil while alive. When plants die, the carbon they contain is both returned to the air and transferred to the soil through burning or decomposition by microorganisms. Because of plants’ ability to remove carbon from the atmosphere, conserving and restoring plant communities such as forests and grasslands are important climate change solutions.
Grasslands are estimated to store about one third of the planet’s land carbon. [11] Unlike trees, the biomass of grasses is mostly underground, in their root systems. Tallgrass prairie species can have roots 5 to 15 feet deep, and purple needlegrass (Stipa pulchra), a grass native to California, has roots that can extend 20 feet deep.
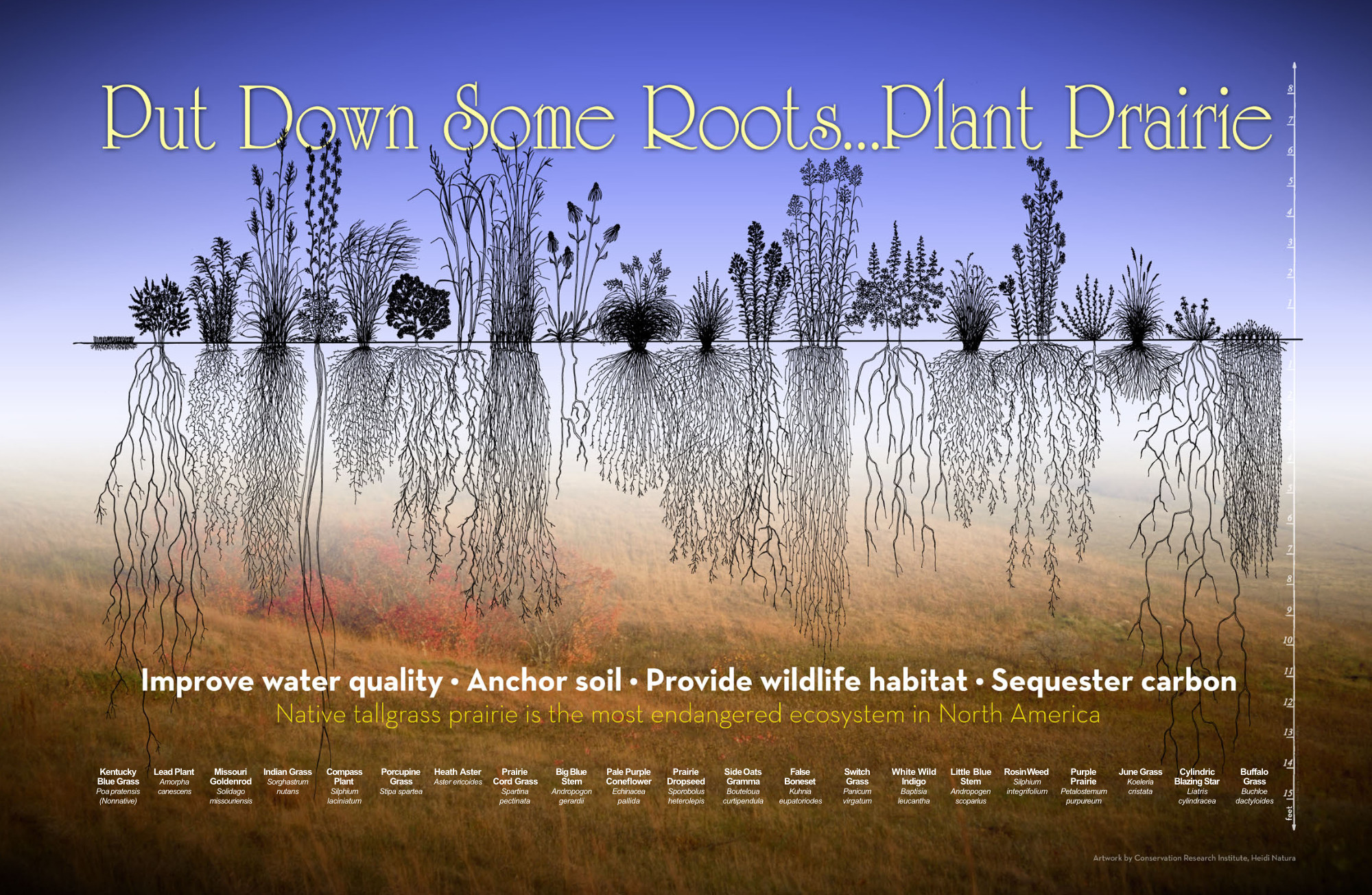
Diagram of plant height and root depth for tallgrass prairie plants native to North America. The height scale goes up to 8 feet and the root scale goes down 15 feet. Artwork by Conservation Research Institute, Heidi Natura. Source: Minnesota Dept. of Natural Resources, https://files.dnr.state.mn.us/assistance/nrplanning/community/roadsidesforwildlife/putdownroots_poster.pdf, accessed Aug. 29, 2023.
About 90% of the carbon stored by grasslands is in roots and soil carbon. [11] This underground storage means that when grasslands burn or are grazed, the carbon they store remains largely intact. Prescribed grassland burns and managed grazing can promote grass growth, leading to increased carbon storage. Both forests and grasslands have a role in carbon storage; author Julia Rosen notes that “grasslands store less carbon per acre than forests, on average, but in a volatile climate, they can often store it longer.” [12]
A study [11] published in 2022 found the following potential for soil organic carbon sequestration in grasslands around the world:
Action
Carbon sequestration rate (billion tons of CO₂eq/year)
Biodiversity restoration
2.3 - 7.3
Improved grazing management
0.148 - 0.699
Sown legumes in pasturelands
0.147
TOTAL
2.6 - 8.1
In comparison, global CO₂ emissions from energy combustion and industrial processes were 36.8 billion tons in 2022. [13]
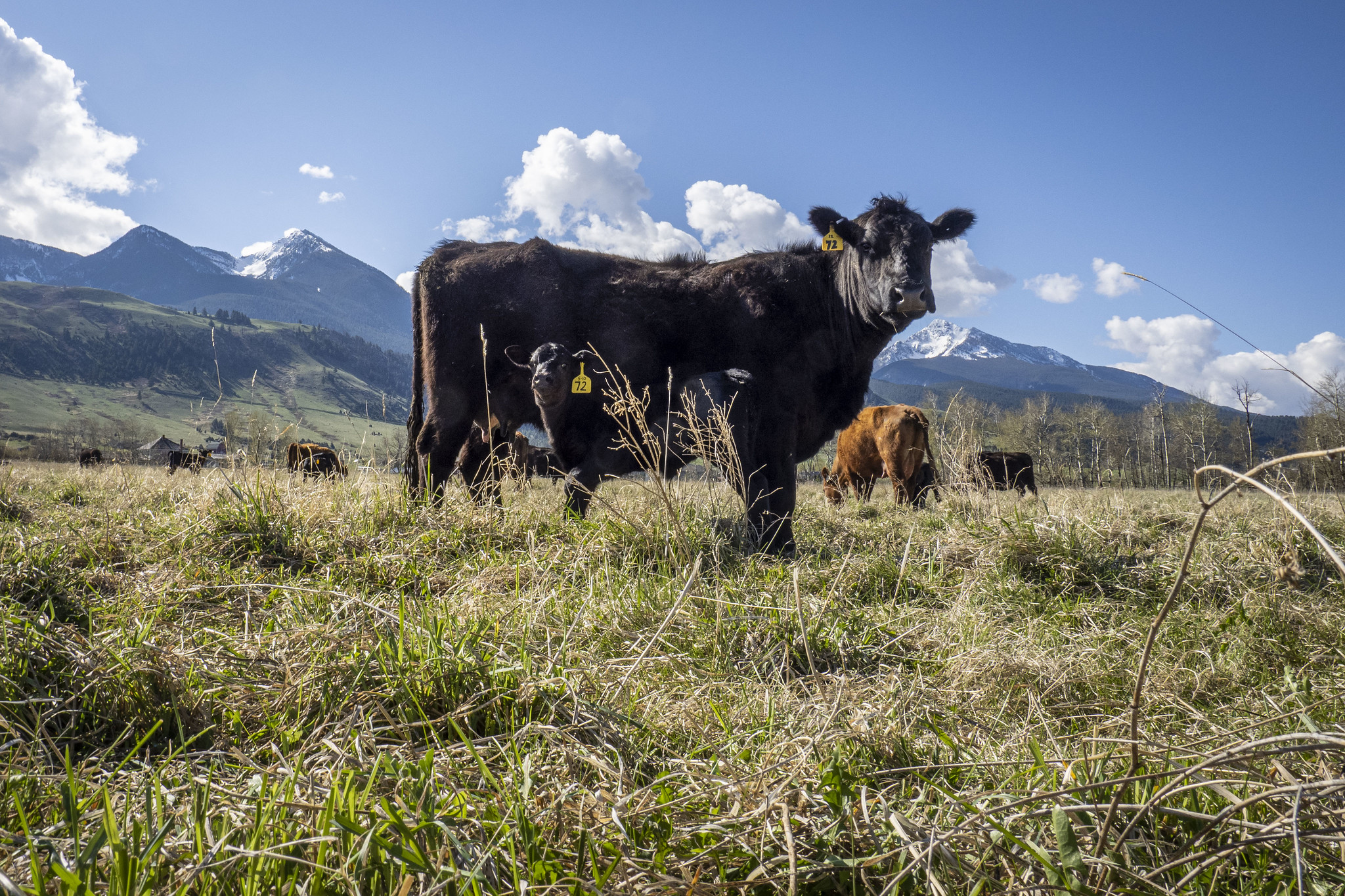
Photo from Pete and Meagan Lannan’s Barney Creek Livestock operation, based on the Jordan Ranch in Livingston, Montana, May 23, 2022. The ranch practices regenerative agriculture in order to promote healthy soil, for example, moving the cows daily to maximize plant recovery after grazing. Photo by Preson Keres, USDA/FPAC (public domain) via Flickr.
BioEnergy with Carbon Capture and Storage (BECCS)
When we burn plant-derived biofuels for heat, electricity, and transportation, we release the CO₂ that was originally taken in by the plant sources of the biofuels. What if we could capture the CO₂ before releasing it, and store it deep underground or use it in industry? Then the process would in principle be carbon emissions-negative, that is, it would result in a net removal of CO₂ from the atmosphere. This is the idea behind BioEnergy with Carbon Capture and Storage, or BECCS.
Forestry and agricultural wastes are often mentioned as potential biofuel sources for BECCS. One example is corn stover—the stalks, cobs, and leaves left in a corn field after the harvest. Another source is plants grown on "marginal" lands—land that is not optimally productive for crops. The table below shows estimates of the productivity of various biofuel sources, including several grasses, in regions of the U.S.
Productivity of Selected Bioenergy Crops by Region of the U.S. (tonnes per hectare)

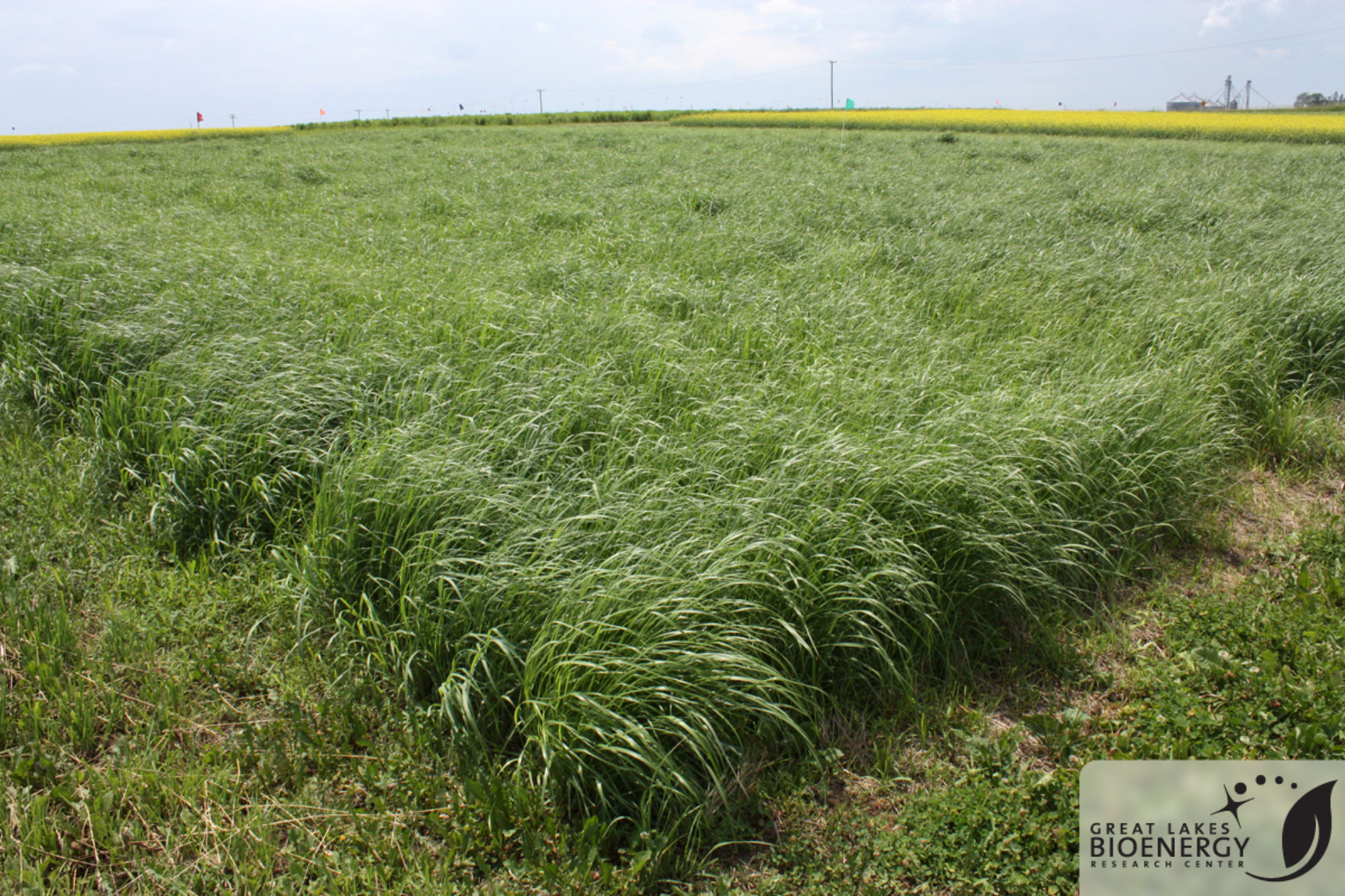
Switchgrass grown for bioenergy research. Photo by Great Lakes Bioenergy Research Center (CC BY-NC-ND 2.0 license) via Flickr.
When compared with other methods of carbon dioxide removal, BECCS is considered to be in the middle range of cost (at an estimated $20 - $100 per tonne of CO₂ removed) and at the high end of potential for removing CO₂. [14] The potential of BECCS to remove CO₂ from the air, given current technology and understanding, is estimated to be between 3.5 and 5.2 billion tonnes per year. [14] Lower cost options include nature-based methods such as conserving and restoring coastal ecosystems that serve as carbon sinks; reforestation and afforestation (planting trees where they did not previously grow); forest management; and agricultural practices that increase soil carbon storage. Higher cost options are direct air capture (using machines to pull CO₂ out of the air) and carbon mineralization (using reactive minerals on land surfaces to react with CO₂ in the air).
Concerns about BECCS include:
- the amount of land needed to grow bioenergy plants if BECCS is implemented on a large scale
- competition for land to grow biofuel sources vs. land for food and fiber crops and grasslands for grazing
- possible destruction of important habitat, and loss of biodiversity
- the efficacy of the method, particularly when fossil fuels are used in biofuel production.
As of 2023, BECCS implementation has been low around the world. According to the International Energy Agency,
"Around 2 Mt [million tonnes] CO2 per year are currently captured from biogenic sources, with less than 1 Mt CO2 stored in dedicated storage. Around 90% is captured in bioethanol facilities, one of the lowest-cost BECCS applications due to the high concentration of CO2 in the process gas stream. The largest operating BECCS project to date is the Illinois Industrial CCS Project, which has been capturing CO2 for permanent storage in a deep geological formation since 2018." [15]
The International Energy Agency also notes reasons why interest in BECCS remains high, despite the potential downsides of the method:
"Bioenergy with carbon capture and storage is the only carbon dioxide removal technique that can also provide energy. Because bioenergy can provide high-temperature heat and fuels that work in existing engines, BECCS plays an important role in decarbonising sectors such as heavy industry, aviation and trucking." [15]
References
- Chapter 12: Conclusions and Recommendations in Rosenzweig, C., W. Solecki, A. DeGaetano, M. O'Grady, S. Hassol, P. Grabhorn (Eds.). 2011. Responding to Climate Change in New York State: The ClimAID Integrated Assessment for Effective Climate Change Adaptation.Technical Report (accessed August 9, 2023). New York State Energy Research and Development Authority (NYSERDA), Albany, New York.
- Bezner Kerr, R., T. Hasegawa, R. Lasco, I. Bhatt, D. Deryng, A. Farrell, H. Gurney-Smith, H. Ju, S. Lluch-Cota, F. Meza, G. Nelson, H. Neufeldt, and P. Thornton, 2022: Food, Fibre, and Other Ecosystem Products. In: Climate Change 2022: Impacts, Adaptation and Vulnerability. Contribution of Working Group II to the Sixth Assessment Report of the Intergovernmental Panel on Climate Change [H.-O. Pörtner, D.C. Roberts, M. Tignor, E.S. Poloczanska, K. Mintenbeck, A. Alegría, M. Craig, S. Langsdorf, S. Löschke, V. Möller, A. Okem, B. Rama (eds.)]. Cambridge University Press, Cambridge, UK and New York, NY, USA, pp. 713–906, https://www.ipcc.ch/report/ar6/wg2/chapter/chapter-5/ (accessed Aug. 24, 2023)
- Matiu, M., D.P. Ankerst and A. Menzel, 2017: Interactions between temperature and drought in global and regional crop yield variability during 1961-2014. PLoS ONE, 12 (5), e178339, doi:10.1371/journal.pone.0178339
- Jiang, X. et al. Projected Future Changes in Vegetation in Western North America in the Twenty-First Century. Journal of Climate 26, 3671–3687 (2013). DOI: https://doi.org/10.1175/JCLI-D-12-00430.1
- Rehfeldt, G. E., Crookston, N. L., Sáenz-Romero, C. & Campbell, E. M. North American vegetation model for land-use planning in a changing climate: a solution to large classification problems. Ecological Applications 22, 119–141 (2012). https://doi.org/10.1890/11-0495.1
- Bagne, Karen, Paulette Ford, and Matt Reeves, Grasslands and Climate Change, USDA U.S. Forest Service. https://www.fs.usda.gov/ccrc/topics/grasslands-and-climate-change
-
Kulmatiski, A. & Beard, K. H. Woody plant encroachment facilitated by increased precipitation intensity. Nature Clim Change 3, 833–837 (2013). https://www.nature.com/articles/nclimate1904
-
Augustine, D. J. et al. Elevated CO2 induces substantial and persistent declines in forage quality irrespective of warming in mixedgrass prairie. Ecological Applications 28, 721–735 (2018). https://doi.org/10.1002/eap.1680
-
Havrilla, C. A., Bradford, J. B., Yackulic, C. B. & Munson, S. M. Divergent climate impacts on C3 versus C4 grasses imply widespread 21st century shifts in grassland functional composition. Diversity and Distributions 29, 379–394 (2023). https://doi.org/10.1111/ddi.13669
- IPCC, 2018: Summary for Policymakers. In: Global Warming of 1.5°C. An IPCC Special Report on the impacts of global warming of 1.5°C above pre-industrial levels and related global greenhouse gas emission pathways, in the context of strengthening the global response to the threat of climate change, sustainable development, and efforts to eradicate poverty [Masson-Delmotte, V., P. Zhai, H.-O. Pörtner, D. Roberts, J. Skea, P.R. Shukla, A. Pirani, W. Moufouma-Okia, C. Péan, R. Pidcock, S. Connors, J.B.R. Matthews, Y. Chen, X. Zhou, M.I. Gomis, E. Lonnoy, T. Maycock, M. Tignor, and T. Waterfield (eds.)]. Cambridge University Press, Cambridge, UK and New York, NY, USA, pp. 3-24, https://www.ipcc.ch/sr15/
- Bai, Y. & Cotrufo, M. F. Grassland soil carbon sequestration: Current understanding, challenges, and solutions. Science 377, 603–608 (2022). DOI: 10.1126/science.abo2380
- Rosen, J. Trees Are Overrated. The Atlantic https://www.theatlantic.com/science/archive/2022/07/climate-change-tree-planting-preserve-grass-grasslands/670583/ (2022).
- IEA (2023), CO2 Emissions in 2022, IEA, Paris https://www.iea.org/reports/co2-emissions-in-2022, accessed Aug. 29, 2023
- National Academies of Sciences, Engineering, and Medicine. 2019. Negative Emissions Technologies and Reliable Sequestration: A Research Agenda. Washington, DC: The National Academies Press. https://doi.org/10.17226/25259.
-
Bioenergy with Carbon Capture and Storage - Energy System. IEA https://www.iea.org/energy-system/carbon-capture-utilisation-and-storage/bioenergy-with-carbon-capture-and-storage, accessed Aug. 31, 2023.
Resources
Articles & reports
Bezner Kerr, R., T. Hasegawa, R. Lasco, I. Bhatt, D. Deryng, A. Farrell, H. Gurney-Smith, H. Ju, S. Lluch-Cota, F. Meza, G. Nelson, H. Neufeldt, and P. Thornton, 2022: Food, Fibre, and Other Ecosystem Products. In: Climate Change 2022: Impacts, Adaptation and Vulnerability. Contribution of Working Group II to the Sixth Assessment Report of the Intergovernmental Panel on Climate Change [H.-O. Pörtner, D.C. Roberts, M. Tignor, E.S. Poloczanska, K. Mintenbeck, A. Alegría, M. Craig, S. Langsdorf, S. Löschke, V. Möller, A. Okem, B. Rama (eds.)]. Cambridge University Press, Cambridge, UK and New York, NY, USA, pp. 713–906, doi:10.1017/9781009325844.007.
"Ancient farming strategy holds promise for climate resilience" by Susan Kelley, Cornell Chronicle, January 11, 2023. https://news.cornell.edu/stories/2023/01/ancient-farming-strategy-holds-promise-climate-resilience, accessed Aug. 24, 2023.
Books
Our Changing Menu: Climate Change and the Foods We Love and Need, by Michael P. Hoffmann, Carrie Koplinka-Loehr, and Danielle L. Eiseman, Comstock Publishing Associates (Cornell University Press), Ithaca, NY and London, UK, 2021.
Websites
Project Drawdown—this project presents analyses of many different climate change solutions, including ones related to grasses: